1-Butyl-3-methylimidazolium tetrachloroaluminate
CAS No.: 80432-09-3
Cat. No.: VC3748220
Molecular Formula: C8H15AlCl4N2
Molecular Weight: 308 g/mol
* For research use only. Not for human or veterinary use.
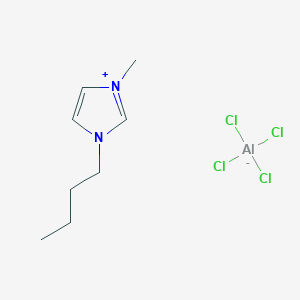
CAS No. | 80432-09-3 |
---|---|
Molecular Formula | C8H15AlCl4N2 |
Molecular Weight | 308 g/mol |
IUPAC Name | 1-butyl-3-methylimidazol-3-ium;tetrachloroalumanuide |
Standard InChI | InChI=1S/C8H15N2.Al.4ClH/c1-3-4-5-10-7-6-9(2)8-10;;;;;/h6-8H,3-5H2,1-2H3;;4*1H/q+1;+3;;;;/p-4 |
Standard InChI Key | PNOZWIUFLYBVHH-UHFFFAOYSA-J |
SMILES | CCCCN1C=C[N+](=C1)C.[Al-](Cl)(Cl)(Cl)Cl |
Canonical SMILES | CCCCN1C=C[N+](=C1)C.[Al-](Cl)(Cl)(Cl)Cl |
Physical and Chemical Properties
Molecular Structure and Composition
1-Butyl-3-methylimidazolium tetrachloroaluminate consists of an organic cation (1-butyl-3-methylimidazolium) and an inorganic anion (tetrachloroaluminate). The molecular formula is C8H15AlCl4N2 with a molecular weight of 308 g/mol. The structure features an imidazolium ring with a methyl group at the 3-position and a butyl chain at the 1-position, while the tetrachloroaluminate anion consists of an aluminum atom coordinated with four chlorine atoms.
Identification Parameters
The compound is identified by several parameters as outlined in Table 1.
Table 1: Identification Parameters of 1-Butyl-3-methylimidazolium Tetrachloroaluminate
Parameter | Value |
---|---|
CAS Number | 80432-09-3 |
Molecular Formula | C8H15AlCl4N2 |
Molecular Weight | 308 g/mol |
VCID | VC3748220 |
CBNumber | CB3441905 |
MDL Number | MFCD06798193 |
Physical Properties
1-Butyl-3-methylimidazolium tetrachloroaluminate typically appears as a viscous liquid at room temperature. Its physical state and properties can vary depending on the purity and exact composition. The compound is classified as a corrosive liquid and should be handled with appropriate safety precautions.
Common Synonyms
The compound is known by several synonyms in the chemical literature and commercial catalogs, as shown in Table 2.
Table 2: Common Synonyms of 1-Butyl-3-methylimidazolium Tetrachloroaluminate
Synonym |
---|
BMIM AlCl4 |
[C4MIm]Cl·AlCl3 |
Basionics AC 01 |
BASIONIC AC 01 |
1-Butyl-3-methylimidazolium chloride and Aluminum chloride (1:2) |
It is important to note that while abbreviations exist in the literature, the full chemical name should be used in formal scientific communication to avoid confusion .
Synthesis Methods
Standard Synthesis Procedure
The synthesis of 1-butyl-3-methylimidazolium tetrachloroaluminate typically involves a two-step process. First, 1-butyl-3-methylimidazolium chloride is prepared, which is then reacted with aluminum chloride hexahydrate. This reaction produces the desired 1-butyl-3-methylimidazolium tetrachloroaluminate in high purity suitable for various applications.
The reaction can be represented as:
-
Preparation of 1-butyl-3-methylimidazolium chloride:
1-methylimidazole + 1-chlorobutane → 1-butyl-3-methylimidazolium chloride -
Formation of tetrachloroaluminate ionic liquid:
1-butyl-3-methylimidazolium chloride + AlCl3 → 1-butyl-3-methylimidazolium tetrachloroaluminate
This synthesis route is preferred for laboratory and industrial preparations due to its relatively straightforward procedure and good yields .
Alternative Synthesis Approaches
Alternative approaches to synthesizing 1-butyl-3-methylimidazolium tetrachloroaluminate may involve different starting materials or reaction conditions. Some researchers have explored modifications to the standard procedure to optimize yield, purity, or to minimize environmental impact.
The molar ratio of AlCl3 to the imidazolium salt is an important parameter that can be adjusted to obtain ionic liquids with different properties. Higher molar ratios of AlCl3 can lead to the formation of Al2Cl7- species, which affects the Lewis acidity of the resulting ionic liquid and consequently its catalytic properties .
Applications
Catalytic Applications
1-Butyl-3-methylimidazolium tetrachloroaluminate has proven to be an effective catalyst for various organic transformations. Its catalytic applications include:
Tetrahydropyranylation Reactions
The compound serves as an efficient catalyst for the tetrahydropyranylation of alcohols, which is an important method for protecting hydroxyl groups in organic synthesis. The ionic liquid facilitates the addition of dihydropyran to alcohols under mild conditions, offering advantages over traditional acid catalysts in terms of selectivity and reusability .
Detetrahydropyranylation Processes
In addition to protection reactions, 1-butyl-3-methylimidazolium tetrachloroaluminate catalyzes the reverse process—detetrahydropyranylation of tetrahydropyranyl (THP) protected ethers. This application is valuable in multi-step organic syntheses where sequential protection and deprotection of functional groups is required .
Alkylation Reactions
The compound has been utilized as a catalyst for the alkylation of benzene with 1-dodecene to synthesize linear alkylbenzenes. This application is industrially significant as linear alkylbenzenes are important precursors for the production of biodegradable detergents .
Extractive Desulfurization of Petroleum Products
One of the most promising applications of 1-butyl-3-methylimidazolium tetrachloroaluminate is in the extractive desulfurization of oil products. The process involves using the ionic liquid to selectively remove sulfur compounds from petroleum products, which is crucial for reducing sulfur emissions and improving fuel quality .
Several studies have investigated the efficiency of this compound in removing sulfur-containing compounds such as thiophene, benzothiophene, and dibenzothiophene from model and real diesel fuels. Research has shown that operational parameters including the volumetric ratio of ionic liquid to fuel, extraction temperature, and contact time significantly influence the desulfurization efficiency .
Table 3 presents typical desulfurization efficiencies reported in the literature under various conditions.
Table 3: Desulfurization Efficiency of 1-Butyl-3-methylimidazolium Tetrachloroaluminate for Different Sulfur Compounds
Sulfur Compound | Initial Concentration (ppm) | Optimal IL:Fuel Ratio | Temperature (°C) | Contact Time (min) | Removal Efficiency (%) |
---|---|---|---|---|---|
Thiophene | 500 | 1:1 | 30 | 30 | 45-60 |
Benzothiophene | 500 | 1:1 | 30 | 30 | 60-75 |
Dibenzothiophene | 500 | 1:1 | 30 | 30 | 75-85 |
Predictive Modeling for Desulfurization
Recent research has focused on developing predictive models for the desulfurization process using 1-butyl-3-methylimidazolium tetrachloroaluminate. A neuro-fuzzy inference system (ANFIS) tuned by particle swarm optimization (PSO) algorithm has been developed to predict sulfur removal from diesel fuel. This model showed good agreement with experimental data and provided better predictions than other modeling approaches .
Mechanistic Studies
Interaction Mechanisms with Sulfur Compounds
Detailed mechanistic studies have been conducted to understand how 1-butyl-3-methylimidazolium tetrachloroaluminate interacts with sulfur compounds during the desulfurization process. Density functional theory (DFT) calculations, using the M06-2X functional combined with implicit solvation models, have provided insights into these interactions .
Dual Functionality in Extractive Desulfurization
One of the key findings from mechanistic studies is that both the cation and anion of 1-butyl-3-methylimidazolium tetrachloroaluminate play important roles in the extractive desulfurization process. The [BMIM]+ cation primarily interacts with aromatic sulfur compounds through π–π interactions, while the [AlCl4]− anion provides hydrogen bonding interactions .
Electrostatic potential analysis suggests that these dominant interactions are driven by electrostatic forces between the ionic liquid and the aromatic sulfur compounds. The dual functionality of the ionic liquid enhances its effectiveness as an extractant for desulfurization processes.
Selectivity Towards Different Sulfur Compounds
Studies have revealed that the interaction energy between 1-butyl-3-methylimidazolium tetrachloroaluminate and various sulfur compounds follows the order: thiophene (TH) < benzothiophene (BT) < dibenzothiophene (DBT). This trend explains the observed selectivity of the ionic liquid in extractive desulfurization processes, where higher removal efficiencies are typically achieved for larger aromatic sulfur compounds .
The higher interaction energy with dibenzothiophene compared to thiophene can be attributed to the increased aromatic surface area, which allows for stronger π–π interactions with the imidazolium cation of the ionic liquid.
Effect of AlCl3 Concentration
The improved desulfurization performance observed with higher molar ratios of AlCl3 is attributed to the Lewis acidity of AlCl3 rather than the formation of [Al2Cl7]− species. This insight is important for optimizing the composition of the ionic liquid for specific desulfurization applications.
Recent Research Developments
Thermal Stability Studies
Recent studies have focused on the thermal stability and degradation pathways of ionic liquids containing the 1-butyl-3-methylimidazolium cation, including the tetrachloroaluminate variant. These investigations have highlighted the complex nature of their decomposition, which involves the formation of various alkyl derivatives of imidazole under different thermal conditions.
Understanding the thermal stability is crucial for applications where the ionic liquid may be exposed to elevated temperatures, such as in high-temperature catalytic processes or thermal recycling procedures.
Advanced Modeling Approaches
Researchers have developed sophisticated modeling approaches to predict the performance of 1-butyl-3-methylimidazolium tetrachloroaluminate in various applications. As mentioned earlier, a neuro-fuzzy inference system tuned by particle swarm optimization has been employed to predict sulfur removal efficiency in desulfurization processes .
These advanced modeling techniques facilitate the optimization of process parameters without extensive experimental work, thus saving time and resources in industrial applications.
Mass Molarity Calculator
- mass of a compound required to prepare a solution of known volume and concentration
- volume of solution required to dissolve a compound of known mass to a desired concentration
- concentration of a solution resulting from a known mass of compound in a specific volume