DL-tert-Leucine
CAS No.: 33105-81-6
Cat. No.: VC21541922
Molecular Formula: C6H13NO2
Molecular Weight: 131.17 g/mol
* For research use only. Not for human or veterinary use.
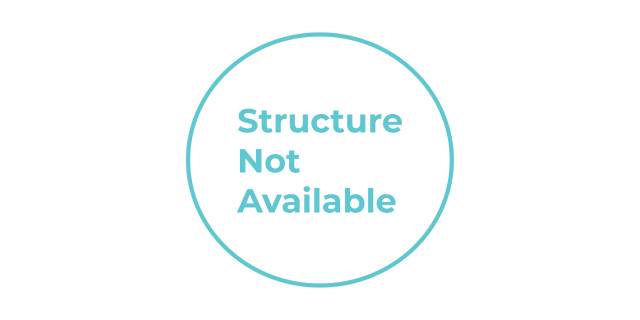
CAS No. | 33105-81-6 |
---|---|
Molecular Formula | C6H13NO2 |
Molecular Weight | 131.17 g/mol |
IUPAC Name | 2-amino-3,3-dimethylbutanoic acid |
Standard InChI | InChI=1S/C6H13NO2/c1-6(2,3)4(7)5(8)9/h4H,7H2,1-3H3,(H,8,9) |
Standard InChI Key | NPDBDJFLKKQMCM-UHFFFAOYSA-N |
SMILES | CC(C)(C)C(C(=O)O)N |
Canonical SMILES | CC(C)(C)C(C(=O)O)N |
Chemical Identity and Properties of DL-tert-Leucine
DL-tert-Leucine (CAS: 33105-81-6) is an unnatural amino acid with the IUPAC name 2-amino-3,3-dimethylbutanoic acid. It exists as a racemic mixture of the L and D stereoisomers. The compound features a tert-butyl group that provides significant hydrophobicity and steric hindrance, making it valuable for controlling the conformation of polypeptide molecules in biosynthesis and pharmaceutical applications .
Physical and Chemical Properties
The compound is characterized by the following properties:
Property | Value |
---|---|
Molecular Formula | C6H13NO2 |
Molecular Weight (g/mol) | 131.18 |
Melting Point | >300°C (decomposition) |
Physical Form | White to off-white solid |
Solubility in Water | 0.1 g/mL, clear, colorless |
SMILES Notation | CC(C)(C)C(N)C(O)=O |
InChI Key | NPDBDJFLKKQMCM-UHFFFAOYSA-N |
Table 1: Physical and chemical properties of DL-tert-Leucine
Alternative Nomenclature
DL-tert-Leucine is known by multiple synonyms in scientific literature and commercial contexts:
-
DL-tert-butylglycine
-
(±)-2-Amino-3,3-dimethylbutyric acid
-
3-methylvaline
-
tert-butylglycine
-
H-DL-Tle-OH
Synthesis Methods for DL-tert-Leucine
The synthesis of DL-tert-Leucine can be accomplished through various chemical and enzymatic methods, each with distinct advantages depending on the scale and intended application.
Chemical Synthesis
DL-tert-Leucine can be prepared using the method described by Jaeger et al. (J. Am. Chem. Soc. 101, 717-732, 1979), which is referenced in multiple patents and scientific publications . This synthetic approach has been utilized as a standard procedure for obtaining the racemic mixture, which can later be resolved to obtain pure enantiomers if required .
Resolution Techniques for Obtaining Pure Enantiomers
Multiple techniques have been developed to resolve DL-tert-Leucine into its pure enantiomers:
Resolution using Dibenzoyl-d-tartaric Acid
One of the most efficient methods involves using dibenzoyl-d-tartaric acid as a resolving agent:
-
DL-tert-Leucine is dissolved in water (chosen for the high solubility of tert-leucine and environmental considerations).
-
The aqueous solution is treated with dibenzoyl-d-tartaric acid (typically 0.5 equivalents).
-
L-tert-Leucine selectively forms a salt with dibenzoyl-d-tartaric acid, which precipitates due to its poor solubility.
-
The diastereomeric salt is filtered and hydrolyzed using mineral acids.
-
The L-tert-Leucine acid salt is neutralized to obtain free L-tert-Leucine .
This method offers several advantages:
-
Direct resolution without requiring derivative formation
-
High recovery of the resolving agent due to solubility differences
Enzymatic Resolution Methods
Several enzymatic approaches have been developed for resolving DL-tert-Leucine:
-
Hog kidney amidase for resolving DL-tert-Leucine amide
-
Penicillin G acylase from Kluyvera citrophila for enantioselective hydrolysis of N-phenylacetylated-DL-tert-Leucine
-
Proteases like Alcalase from Bacillus licheniformis for enzymatic hydrolysis of (±)-N-acetyl-tert-leucine chloroethyl ester
Enzymatic Synthesis of L-tert-Leucine
Recent research has focused extensively on enzymatic methods for the direct synthesis of L-tert-Leucine, which offer advantages of higher stereoselectivity, milder reaction conditions, and environmental sustainability.
Leucine Dehydrogenase-Catalyzed Synthesis
Leucine dehydrogenase (LeuDH) enzymes can catalyze the reductive amination of trimethylpyruvic acid (TMP) to produce L-tert-Leucine with high enantiomeric purity. These enzymes require NADH as a cofactor, which can be regenerated using formate dehydrogenase (FDH) .
Novel Leucine Dehydrogenases
Various novel leucine dehydrogenases have been identified through genome mining approaches:
Table 2: Sources and characteristics of leucine dehydrogenases for L-tert-Leucine synthesis
Kinetic Parameters and Substrate Specificity
The enzymatic properties of leucine dehydrogenases significantly influence their effectiveness in L-tert-Leucine synthesis. Kinetic studies of EsiLeuDH from Exiguobacterium sibiricum revealed the following parameters:
Substrate | Km (mM) | Vmax (μmol·min⁻¹·mg⁻¹) | kcat (s⁻¹) | kcat/Km (L·mmol⁻¹·s⁻¹) |
---|---|---|---|---|
NADH | 0.624 | 114.16 | 76.86 | 123.17 |
TMP | 5.96 | 67.48 | 45.43 | 7.62 |
NAD+ | 1.46 | 12.49 | 8.41 | 5.76 |
L-Leucine | 0.88 | 6.4 | 4.31 | 4.90 |
Table 3: Kinetic parameters of EsiLeuDH for various substrates
The lower Km value for TMP (5.96 mM) compared to other reported leucine dehydrogenases (16.8-25.7 mM) indicates a higher affinity for the substrate, making EsiLeuDH a more efficient catalyst for L-tert-Leucine synthesis .
Process Optimization for Large-Scale Production
Several factors affect the efficiency of enzymatic L-tert-Leucine production:
Effect of Substrate Concentration
TMP (M) | EsiLeuDH (g·L⁻¹) | FDH (g·L⁻¹) | Reaction Time (h) | Conversion (%) | Yield (%) |
---|---|---|---|---|---|
0.2 | 10 | 20 | 6 | 98 | 66 |
0.3 | 10 | 20 | 10 | 99 | 61 |
0.4 | 10 | 20 | 12 | 98 | 80 |
0.5 | 10 | 20 | 24 | 98 | 78 |
0.6 | 10 | 20 | 24 | 83 | 64 |
0.5 | 20 | 30 | 20 | 98 | 96 |
0.5 | 20 | 40 | 12 | 98 | 80 |
Table 4: Effect of substrate and enzyme concentrations on L-tert-Leucine production
As TMP concentration increases, longer reaction times are required, and at high concentrations (0.6 M), substrate inhibition reduces conversion rates. Higher enzyme loadings can mitigate this effect and improve yields .
Fed-Batch Strategy
To overcome substrate inhibition, fed-batch strategies have been developed where TMP is added incrementally to maintain concentrations below 40 mM. This approach has achieved L-tert-Leucine concentrations of 65.6 g·L⁻¹ with average conversion rates of 81% after 30 hours .
Bifunctional Enzyme Complexes
Advanced enzyme engineering approaches have developed bifunctional enzyme complexes where leucine dehydrogenase and formate dehydrogenase are assembled on a miniscaffoldin. This arrangement enhances the initial L-tert-Leucine production rate by 2-fold compared to free enzyme mixtures, enabling production of 91 g·L⁻¹ of L-tert-Leucine with 99% enantiomeric purity .
Applications of DL-tert-Leucine and its Enantiomers
DL-tert-Leucine and its resolved enantiomers have diverse applications across multiple industries and research areas.
Pharmaceutical Applications
L-tert-Leucine serves as a critical intermediate in the synthesis of numerous pharmaceuticals:
The unique tert-butyl group creates significant hydrophobicity and steric hindrance that can effectively control the conformation of polypeptide molecules, enhancing pharmacological properties .
Applications in Asymmetric Synthesis
Both DL-tert-Leucine and its resolved enantiomers serve as valuable chiral auxiliaries in organic synthesis:
-
L-tert-Leucine derivatives, such as thiazolidinethione auxiliaries, enable highly diastereoselective acetate aldol reactions with dichlorophenylborane
-
As building blocks for chiral catalysts in asymmetric transformations
-
In the preparation of enantiomerically pure compounds for pharmaceutical and fine chemical industries
Additional Industrial Applications
Beyond pharmaceutical and chemical synthesis applications:
-
Animal feed additives
-
Nutritional fortifiers in food products
-
Cosmetic ingredients
-
Templates for inducing asymmetric synthesis with high stereoselectivity
Recent Research Developments
Recent scientific advancements related to DL-tert-Leucine have focused on improving synthesis efficiency, enzyme engineering, and novel applications.
Enzyme Engineering and Biocatalyst Enhancement
Researchers have made significant progress in developing more efficient biocatalysts:
-
Self-purification and self-assembly coenzyme regeneration systems
-
Bifunctional enzyme complexes based on cohesin-dockerin interactions
-
Genome mining strategies to identify novel leucine dehydrogenases with enhanced catalytic properties
Analytical Methods
Advanced analytical techniques have been developed for determining the enantiomeric purity of tert-Leucine products:
-
HPLC analysis using Thermo Hypersil BDS C18 columns with 2-O-acetyl-β-D-glucopyranose isothiocyanate (GITC) as a derivatization reagent
-
Detection at 254 nm wavelength with methanol:phosphate buffer (58:42) as the mobile phase
These methods enable accurate determination of enantiomeric excess values, often achieving 99.99% e.e. for enzymatically produced L-tert-Leucine .
Challenges and Future Prospects
Despite significant advances, several challenges remain in the production and application of DL-tert-Leucine and its enantiomers.
Technical Challenges
-
Overcoming substrate inhibition at high concentrations
-
Improving enzyme stability and reusability
-
Developing more cost-effective cofactor regeneration systems
Future Research Directions
Promising areas for future research include:
-
Development of continuous flow processes for large-scale enzymatic synthesis
-
Protein engineering to enhance enzyme thermostability and catalytic efficiency
-
Expanded applications in peptide drug design and pharmaceutical synthesis
-
Novel immobilization techniques for enhanced enzyme recovery and reuse
Mass Molarity Calculator
- mass of a compound required to prepare a solution of known volume and concentration
- volume of solution required to dissolve a compound of known mass to a desired concentration
- concentration of a solution resulting from a known mass of compound in a specific volume