D-beta-homoserine
CAS No.: 16504-57-7
Cat. No.: VC21540921
Molecular Formula: C4H9NO3
Molecular Weight: 119,12 g/mole
* For research use only. Not for human or veterinary use.
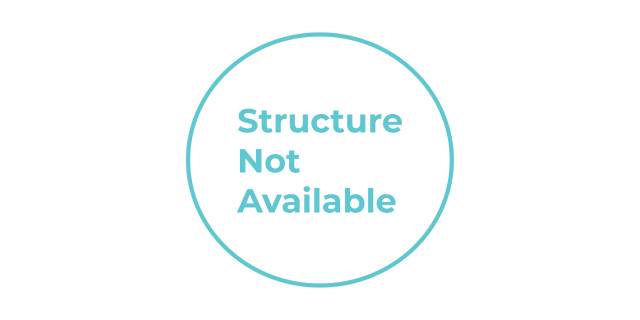
CAS No. | 16504-57-7 |
---|---|
Molecular Formula | C4H9NO3 |
Molecular Weight | 119,12 g/mole |
IUPAC Name | (3S)-3-amino-4-hydroxybutanoic acid |
Standard InChI | InChI=1S/C4H9NO3/c5-3(2-6)1-4(7)8/h3,6H,1-2,5H2,(H,7,8)/t3-/m0/s1 |
Standard InChI Key | BUZICZZQJDLXJN-VKHMYHEASA-N |
Isomeric SMILES | C([C@@H](CO)N)C(=O)O |
SMILES | C(C(CO)N)C(=O)O |
Canonical SMILES | C(C(CO)N)C(=O)O |
Chemical Structure and Properties
D-beta-homoserine is an amino acid with the molecular formula C4H9NO3 and a molecular weight of 119.12 g/mol. It is characterized by a specific stereochemical configuration at the chiral carbon atom, which distinguishes it from its L-enantiomer. The compound features a primary amino group (-NH2), a hydroxyl group (-OH), and a carboxylic acid group (-COOH) arranged along a four-carbon backbone.
Physical Properties
D-beta-homoserine typically appears as a white to light yellowish crystalline powder, though variations in color from white to orange or green may occur depending on purity and storage conditions . Its physical properties include:
Property | Value |
---|---|
Melting Point | 205°C (with decomposition) |
Boiling Point | 368.7±32.0°C (Predicted) |
Density | 1.312±0.06 g/cm³ (Predicted) |
Optical Rotation | 8.0° (C=2, H₂O) |
pKa | 2.21±0.10 (Predicted) |
LogP | -1.289 (estimated) |
Solubility Profile
D-beta-homoserine demonstrates good solubility in various solvents, making it versatile for laboratory applications. It is readily soluble in water and also dissolves in organic solvents including:
This solubility profile facilitates its use in diverse experimental settings and synthetic applications.
Synthesis and Production Methods
Biological Production
Homoserine compounds are often produced through microbial fermentation processes. Research has demonstrated that engineered strains of Escherichia coli can be utilized for the efficient production of homoserine from glucose. Recent studies have reported significant improvements in production metrics, with one study achieving:
Further advancements have pushed these numbers even higher:
These improvements were achieved through metabolic engineering approaches that divided the pathway into three modules:
-
Upstream: glucose transport and glycolysis
-
Midstream: TCA and glyoxylate cycles
-
Downstream: conversion of aspartate to homoserine and its secretion
While these studies focused on L-homoserine production, similar principles could potentially be applied to D-beta-homoserine production with appropriate adjustments for stereoselectivity.
Biological Functions
Metabolic Role
D-beta-homoserine plays significant roles in various biochemical pathways. Homoserine compounds generally serve as intermediates in the biosynthesis of essential amino acids, including methionine, threonine, and isoleucine. They participate in enzymatic reactions catalyzed by homoserine dehydrogenase and related enzymes.
Microbial Utilization
Some bacteria can utilize homoserine compounds as sole carbon and nitrogen sources. The degradation of homoserine in microorganisms involves specialized enzymes, including homoserine dehydrogenase. A study on Arthrobacter nicotinovorans identified a novel homoserine dehydrogenase (AnHSD) specifically responsible for L-homoserine catabolism . This enzyme shows high specificity for homoserine degradation and demonstrates a preference for NAD+ as a cofactor.
While this specific research focused on L-homoserine, it provides context for understanding how D-beta-homoserine might be metabolized in biological systems.
Applications in Research and Industry
Bacterial Polysaccharide Synthesis
D-beta-homoserine is utilized in the synthesis of bacterial polysaccharides, notably the O-antigen of Acinetobacter lwoffii EK30A . These bacterial polysaccharides are crucial components of cell walls and have significant implications for bacterial virulence, immune recognition, and potential therapeutic applications.
Enzyme Mechanism Studies
Another important application of D-beta-homoserine is in the production of atypical serine proteases for mechanism studies . These studies contribute to our understanding of enzyme structure-function relationships and catalytic mechanisms, with potential applications in biotechnology and pharmaceutical development.
Comparison with Structurally Related Compounds
L-homoserine vs. D-beta-homoserine
L-homoserine and D-beta-homoserine are stereoisomers, differing in the spatial arrangement of their functional groups. L-homoserine is more commonly found in biological systems as an intermediate in amino acid biosynthesis pathways. In contrast, D-beta-homoserine's unique stereochemistry may confer different biological activities and applications.
Homoserine Lactones
Homoserine lactones, particularly N-acyl-homoserine lactones, are cyclic derivatives formed when the hydroxyl group of homoserine forms an internal ester with the carboxyl group. These compounds serve as important signaling molecules in bacterial quorum sensing systems . The structural relationship between homoserine lactones and D-beta-homoserine suggests potential for the latter in studies of bacterial communication mechanisms.
Analytical Methods for Detection and Quantification
Chromatographic Techniques
Thin-layer chromatography (TLC) has been employed for the separation and quantification of homoserine compounds and derivatives. In one study, laser densitometry was used to quantify N-acyl-homoserine lactones separated by TLC, with the response being linear over at least two orders of magnitude . Similar techniques could potentially be applied to D-beta-homoserine analysis.
Mass Spectrometry
Mass spectrometry provides a powerful tool for the characterization of homoserine compounds. Tandem mass spectrometry (MS/MS) can be particularly useful for structural confirmation, as demonstrated in studies of homoserine lactones where fragmentation patterns revealed characteristic ions associated with the homoserine lactone moiety .
Current Research Trends and Future Perspectives
Metabolic Engineering for Production
Recent advances in metabolic engineering have significantly improved the production of homoserine compounds in microbial systems. Strategies such as:
-
Overexpression of key enzymes in the biosynthetic pathway
-
Attenuation of competing pathways
-
Balancing of carbon fluxes through pathway modules
-
Engineering of export systems for improved product secretion
These approaches have led to substantial improvements in titer, yield, and productivity . Continuing research in this area may lead to more efficient production methods for D-beta-homoserine and related compounds.
Novel Enzyme Discovery
The identification of specialized enzymes involved in homoserine metabolism, such as the novel homoserine dehydrogenase from Arthrobacter nicotinovorans, opens new avenues for understanding how homoserine compounds are processed in biological systems . Further research into enzymes specifically acting on D-beta-homoserine could reveal unique metabolic pathways and potential applications.
Mass Molarity Calculator
- mass of a compound required to prepare a solution of known volume and concentration
- volume of solution required to dissolve a compound of known mass to a desired concentration
- concentration of a solution resulting from a known mass of compound in a specific volume