Boc-N-ME-phg-OH
CAS No.: 30925-11-2
VCID: VC21540687
Molecular Formula: C14H19NO4
Molecular Weight: 265,31 g/mole
* For research use only. Not for human or veterinary use.
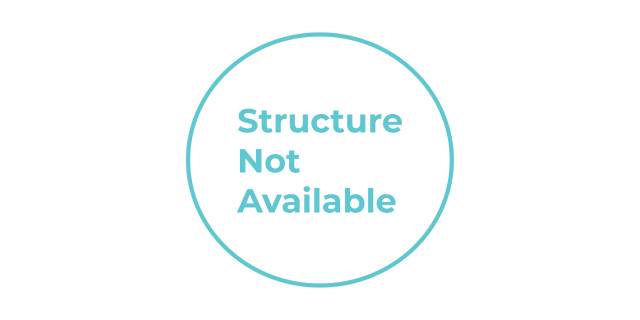
Description |
Boc-N-ME-phg-OH, also known as N-tert-butoxycarbonyl-N-methyl-phenylglycine, is a protected amino acid derivative commonly used in peptide synthesis. It is available in both D- and L- forms, with the D-form being Boc-D-N-ME-phg-OH and the L-form being Boc-N-Me-Phg-OH. The compound is crucial for incorporating N-methylated amino acids into peptides, which can significantly affect the biological activity and stability of the resulting peptides. Synthesis and ApplicationsBoc-N-ME-phg-OH is synthesized through the protection of N-methyl-phenylglycine with a Boc group. This process typically involves reacting N-methyl-phenylglycine with di-tert-butyl dicarbonate (Boc2O) in the presence of a base like triethylamine or pyridine. Applications:
Research FindingsResearch on Boc-N-ME-phg-OH and similar compounds highlights their importance in peptide chemistry. For instance, the use of Boc-protected amino acids facilitates the synthesis of complex peptides by protecting the amino group during coupling reactions, thereby preventing unwanted side reactions . Biological SignificanceN-methylated peptides can exhibit altered biological activities compared to their non-methylated counterparts. This modification can affect the binding affinity to receptors or enzymes, potentially leading to improved therapeutic properties . Chemical StabilityThe Boc group is known for its stability under various conditions but can be easily removed under acidic conditions, such as treatment with trifluoroacetic acid (TFA), to reveal the free amine . Synthesis Conditions
Applications
|
||||||||||
---|---|---|---|---|---|---|---|---|---|---|---|
CAS No. | 30925-11-2 | ||||||||||
Product Name | Boc-N-ME-phg-OH | ||||||||||
Molecular Formula | C14H19NO4 | ||||||||||
Molecular Weight | 265,31 g/mole | ||||||||||
IUPAC Name | (2S)-2-[methyl-[(2-methylpropan-2-yl)oxycarbonyl]amino]-2-phenylacetic acid | ||||||||||
Standard InChI | InChI=1S/C14H19NO4/c1-14(2,3)19-13(18)15(4)11(12(16)17)10-8-6-5-7-9-10/h5-9,11H,1-4H3,(H,16,17)/t11-/m0/s1 | ||||||||||
Standard InChIKey | COABPHLHHQAKPL-NSHDSACASA-N | ||||||||||
Isomeric SMILES | CC(C)(C)OC(=O)N(C)[C@@H](C1=CC=CC=C1)C(=O)O | ||||||||||
SMILES | CC(C)(C)OC(=O)N(C)C(C1=CC=CC=C1)C(=O)O | ||||||||||
Canonical SMILES | CC(C)(C)OC(=O)N(C)C(C1=CC=CC=C1)C(=O)O | ||||||||||
Synonyms | BOC-N-ME-PHG-OH;30925-11-2;(S)-2-((TERT-BUTOXYCARBONYL)(METHYL)AMINO)-2-PHENYLACETICACID;AmbotzBAA1259;PubChem12248;SCHEMBL994016;MolPort-006-701-270;EBD54295;ZINC2391142;AKOS016001117;CB-1687;AJ-35729;AK-49669;SC-22507;KB-276686;FT-0638943;ST24046234;K-7373;(S)-[Methyl(tert-butoxycarbonyl)amino]phenylaceticacid;(2S)-[(tert-Butoxycarbonyl)(methyl)amino](phenyl)aceticacid;(S)-[(tert-butoxycarbonyl)(methyl)amino](phenyl)aceticacid | ||||||||||
PubChem Compound | 7010620 | ||||||||||
Last Modified | Aug 15 2023 |
Mass Molarity Calculator
- mass of a compound required to prepare a solution of known volume and concentration
- volume of solution required to dissolve a compound of known mass to a desired concentration
- concentration of a solution resulting from a known mass of compound in a specific volume