Sirolimus, also known as rapamycin, is a macrocyclic lactone antibiotic produced by bacteria Streptomyces hygroscopicus, which was isolated from the soil of the Vai Atari region of Rapa Nui (Easter Island). It was first isolated and identified as an antifungal agent with potent anticandida activity; however, after its potent antitumor and immunosuppressive activities were later discovered, it was extensively investigated as an immunosuppressive and antitumour agent. Its primary mechanism of action is the inhibition of the mammalian target of rapamycin (mTOR), which is a serine/threonine-specific protein kinase that regulates cell growth, proliferation, and survival. mTOR is an important therapeutic target for various diseases, as it was shown to regulate longevity and maintain normal glucose homeostasis. Targeting mTOR received more attention especially in cancer, as mTOR signalling pathways are constitutively activated in many types of human cancer. Sirolimus was first approved by the FDA in 1999 for the prophylaxis of organ rejection in patients aged 13 years and older receiving renal transplants. In November 2000, the drug was recognized by the European Agency as an alternative to calcineurin antagonists for maintenance therapy with corticosteroids. In May 2015, the FDA approved sirolimus for the treatment of patients with lymphangioleiomyomatosis. In November 2021, albumin-bound sirolimus for intravenous injection was approved by the FDA for the treatment of adults with locally advanced unresectable or metastatic malignant perivascular epithelioid cell tumour (PEComa). Sirolimus was also investigated in other cancers such as skin cancer, Kaposi’s Sarcoma, cutaneous T-cell lymphomas, and tuberous sclerosis. The topical formulation of sirolimus, marketed as HYFTOR, was approved by the FDA in April 2022: this marks the first topical treatment approved in the US for facial angiofibroma associated with tuberous sclerosis complex.
Sirolimus is a mTOR Inhibitor Immunosuppressant and Kinase Inhibitor. The mechanism of action of sirolimus is as a mTOR Inhibitor and Protein Kinase Inhibitor. The physiologic effect of sirolimus is by means of Decreased Immunologic Activity.
Sirolimus is macrocyclic antibiotic with potent immunosuppressive activity that is used alone or in combination with calcineurin inhibitors and corticosteroids to prevent cellular rejection after renal transplantation. Sirolimus therapy can be associated with mild serum enzyme elevations and it has been linked to rare instances of clinically apparent cholestatic liver injury.
Sirolimus is a natural product found in Streptomyces iranensis, Streptomyces rapamycinicus, and other organisms with data available.
Sirolimus is a natural macrocyclic lactone produced by the bacterium Streptomyces hygroscopicus, with immunosuppressant properties. In cells, sirolimus binds to the immunophilin FK Binding Protein-12 (FKBP-12) to generate an immunosuppressive complex that binds to and inhibits the activation of the mammalian Target Of Rapamycin (mTOR), a key regulatory kinase. This results in inhibition of T lymphocyte activation and proliferation that occurs in response to antigenic and cytokine (IL-2, IL-4, and IL-15) stimulation and inhibition of antibody production. (NCI04)
A macrolide compound obtained from Streptomyces hygroscopicus that acts by selectively blocking the transcriptional activation of cytokines thereby inhibiting cytokine production. It is bioactive only when bound to IMMUNOPHILINS. Sirolimus is a potent immunosuppressant and possesses both antifungal and antineoplastic properties.
Rapamycin
CAS No.: 53123-88-9
Cat. No.: VC21540458
Molecular Formula: C51H79NO13
Molecular Weight: 914.2 g/mol
Purity: >98% (or refer to the Certificate of Analysis)
* For research use only. Not for human or veterinary use.
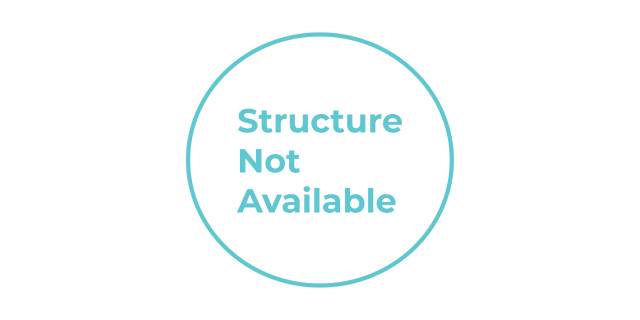
CAS No. | 53123-88-9 |
---|---|
Molecular Formula | C51H79NO13 |
Molecular Weight | 914.2 g/mol |
IUPAC Name | (1R,9S,12S,15R,16E,18R,19R,21R,23S,24E,26E,28E,30S,32S,35R)-1,18-dihydroxy-12-[(2R)-1-[(1S,3R,4R)-4-hydroxy-3-methoxycyclohexyl]propan-2-yl]-19,30-dimethoxy-15,17,21,23,29,35-hexamethyl-11,36-dioxa-4-azatricyclo[30.3.1.04,9]hexatriaconta-16,24,26,28-tetraene-2,3,10,14,20-pentone |
Standard InChI | InChI=1S/C51H79NO13/c1-30-16-12-11-13-17-31(2)42(61-8)28-38-21-19-36(7)51(60,65-38)48(57)49(58)52-23-15-14-18-39(52)50(59)64-43(33(4)26-37-20-22-40(53)44(27-37)62-9)29-41(54)32(3)25-35(6)46(56)47(63-10)45(55)34(5)24-30/h11-13,16-17,25,30,32-34,36-40,42-44,46-47,53,56,60H,14-15,18-24,26-29H2,1-10H3/b13-11+,16-12+,31-17+,35-25+/t30-,32-,33-,34-,36-,37+,38+,39+,40-,42+,43+,44-,46-,47+,51-/m1/s1 |
Standard InChI Key | QFJCIRLUMZQUOT-HPLJOQBZSA-N |
Isomeric SMILES | C[C@@H]1CC[C@H]2C[C@@H](/C(=C/C=C/C=C/[C@H](C[C@H](C(=O)[C@@H]([C@@H](/C(=C/[C@H](C(=O)C[C@H](OC(=O)[C@@H]3CCCCN3C(=O)C(=O)[C@@]1(O2)O)[C@H](C)C[C@@H]4CC[C@H]([C@@H](C4)OC)O)C)/C)O)OC)C)C)/C)OC |
SMILES | CC1CCC2CC(C(=CC=CC=CC(CC(C(=O)C(C(C(=CC(C(=O)CC(OC(=O)C3CCCCN3C(=O)C(=O)C1(O2)O)C(C)CC4CCC(C(C4)OC)O)C)C)O)OC)C)C)C)OC |
Canonical SMILES | CC1CCC2CC(C(=CC=CC=CC(CC(C(=O)C(C(C(=CC(C(=O)CC(OC(=O)C3CCCCN3C(=O)C(=O)C1(O2)O)C(C)CC4CCC(C(C4)OC)O)C)C)O)OC)C)C)C)OC |
Appearance | White to off-white solid powder |
Boiling Point | 183-185 |
Colorform | Colorless crystalline solid from ether |
Melting Point | 183-185 °C |
Chemical Structure and Properties
Rapamycin is a complex macrocyclic lactone with molecular formula C51H79NO13 and molecular weight of 914.2 g/mol . Its structure is characterized by a large 31-membered macrocyclic ring formed through polyketide synthesis . The molecule's IUPAC name is (1R,9S,12S,15R,16E,18R,19R,21R,23S,24E,26E,28E,30S,32S,35R)-1,18-dihydroxy-12-[(2R)-1-[(1S,3R,4R)-4-hydroxy-3-methoxycyclohexyl]propan-2-yl]-19,30-dimethoxy-15,17,21,23,29,35-hexamethyl-11,36-dioxa-4-azatricyclo[30.3.1.04,9]hexatriaconta-16,24,26,28-tetraene-2,3,10,14,20-pentone .
Key Structural Features
The macrocyclic structure of rapamycin contains several defining characteristics:
-
Multiple ester linkages that stabilize the macrocycle and serve as sites for enzymatic cleavage
-
Several hydroxyl groups that contribute to polarity and facilitate molecular interactions
-
Multiple chiral centers that define its stereochemistry and biological activity
-
A series of double bonds (C=C) interspersed throughout the structure, enhancing hydrophobicity
-
A pipecolate moiety (derived from pipecolic acid) that is critical for target binding
Physical and Chemical Properties
Property | Value |
---|---|
Molecular Formula | C51H79NO13 |
Molecular Weight | 914.18 g/mol |
Physical Appearance | White solid |
Solubility | Soluble to 100 mM in ethanol, 50 mM in DMSO |
Melting Point | 183-185°C |
Storage Temperature | -20°C |
CAS Number | 53123-88-9 |
Table 1: Physical and chemical properties of rapamycin
Discovery and Historical Context
Rapamycin was first isolated in 1972 from a soil sample collected on Rapa Nui (Easter Island) from the bacterium Streptomyces hygroscopicus . Initially investigated as an antifungal agent, researchers soon discovered its potent immunosuppressive properties . This discovery ultimately led to its development as a pharmaceutical agent for preventing organ rejection in transplant recipients.
The compound's name "Rapamycin" derives from "Rapa Nui," the indigenous name for Easter Island, highlighting the increasingly rare instance of a major pharmaceutical compound discovered from natural sources . In 1999, the FDA approved rapamycin (under the brand name Rapamune) for preventing rejection in kidney transplantation, marking the beginning of its clinical use .
Biosynthesis
The biosynthesis of rapamycin occurs naturally in Streptomyces hygroscopicus through a complex pathway involving both polyketide synthase (PKS) and nonribosomal peptide synthetase (NRPS) enzymes .
Biosynthetic Pathway
The biosynthetic process involves several key steps:
-
The core polyketide chain is assembled by three multienzymes: RapA, RapB, and RapC, containing a total of 14 modules
-
The linear polyketide undergoes modification by the NRPS RapP, which attaches L-pipecolate to the terminal end
-
The molecule is then cyclized to form prerapamycin, the first enzyme-free product
-
Further modifications by five enzymes (RapI, RapJ, RapM, RapN, and RapQ) through methylations and oxidations yield the final rapamycin structure
Genetic Basis of Rapamycin Biosynthesis
The biosynthetic genes responsible for rapamycin production include:
-
rapA, rapB, and rapC - encode the multienzymes that assemble the polyketide backbone
-
rapL - encodes a NAD+-dependent lysine cycloamidase that converts L-lysine to L-pipecolic acid
-
rapP - encodes the NRPS responsible for incorporating L-pipecolic acid and cyclizing prerapamycin
-
rapI, rapJ, rapM, rapN, and rapQ - encode enzymes that modify the macrocyclic core through methylation and oxidation reactions
Mechanism of Action
Rapamycin's primary mechanism of action involves inhibition of the mammalian target of rapamycin (mTOR), a serine/threonine protein kinase that plays a central role in regulating cellular metabolism, growth, and proliferation .
mTOR Complexes and Signaling
mTOR exists in two distinct multi-protein complexes:
-
mTOR Complex 1 (mTORC1): Composed of mTOR, Raptor (regulatory associated protein of mTOR), mLST8, PRAS40, and Deptor
-
mTOR Complex 2 (mTORC2): Composed of mTOR, Rictor (rapamycin-insensitive companion of TOR), mSin1, Protor-1/2, mLST8, and Deptor
While mTORC1 is sensitive to rapamycin inhibition, mTORC2 is generally considered rapamycin-insensitive, although prolonged exposure can affect this complex as well .
Molecular Mechanism
The inhibition process follows these steps:
-
Rapamycin binds to the intracellular protein FKBP12 (FK506 binding protein 12)
-
The FKBP12-rapamycin complex binds to the FRB (FKBP12-rapamycin binding) domain of mTOR
-
This binding causes conformational changes that weaken the interaction between mTOR and Raptor
-
The weakened interaction leads to disintegration of the mTORC1 complex and inhibition of its kinase activity
Downstream Effects
Inhibition of mTORC1 affects several key downstream targets:
-
Reduced phosphorylation of p70S6K (ribosomal protein S6 kinase), leading to decreased protein synthesis
-
Decreased phosphorylation of 4E-BP1 (eukaryotic translation initiation factor 4E-binding protein 1), inhibiting mRNA translation
-
Inhibition of cyclin-dependent kinase (cdk2-cyclin E complex) activity through prevention of p27 decline, causing G1/S cell cycle arrest
-
Increased autophagy through effects on Unc-51-like autophagy-activating kinases
-
Altered lipid metabolism through effects on SREBPs (sterol-responsive element-binding proteins) and Lipin-1
Pharmacokinetics and Pharmacodynamics
Rapamycin exhibits complex pharmacokinetic properties that influence its clinical application and dosing strategies.
Absorption and Distribution
Rapamycin is characterized by:
-
Variable oral bioavailability (approximately 14%)
-
Extensive tissue distribution
-
High plasma protein binding (approximately 95%)
-
Blood-to-plasma ratio of approximately 1:3, indicating concentration in red blood cells
Metabolism and Elimination
Key aspects of rapamycin metabolism include:
-
Primary metabolism via the cytochrome P450 3A4 (CYP3A4) enzyme system
-
Elimination primarily through biliary excretion
Dosing Considerations
A recent study on intermittent rapamycin administration in Alzheimer's disease patients found:
-
Moderate interindividual variability in blood concentrations (coefficient of variation 0.28-0.40)
-
The 48-hour post-dose measurement showed the lowest variability (CoV = 0.28) and strongest correlation with pre-dose levels
-
Weekly dosing achieved blood concentrations below immunosuppressive levels
Clinical Applications
Transplantation
Rapamycin (marketed as Rapamune/Sirolimus) was originally approved for:
-
Prevention of organ rejection in kidney transplantation
-
Use in combination with other immunosuppressants such as calcineurin inhibitors and corticosteroids
Cancer Treatment and Prevention
Rapamycin and its derivatives have shown significant potential in oncology:
-
Preventive effects in multiple cancer models, including p53 mutant mice, Apc mutant animals, and HER-2/neu transgenic mice
-
Direct anti-neoplastic properties, including inhibition of cancer growth, promotion of apoptosis, and modification of host response to tumors
-
Potential for cancer prevention in high-risk human populations, particularly for lung cancer in smokers and former smokers
Aging and Longevity
Rapamycin has demonstrated remarkable anti-aging effects:
-
Extension of maximal lifespan in both genders in mice, making it the first pharmacological agent to achieve this milestone
-
Dose-dependent effects on longevity with minimal side effects at lower doses
-
Prevention or delay of multiple age-related changes in various animal models
-
Reduction of cancer incidence, which is a major cause of death in many animal models
Neurodegenerative Diseases
Emerging research suggests potential benefits in neurological conditions:
-
Neuroprotective effects through regulation of autophagy and reduction of neuroinflammation
-
Potential treatment for Alzheimer's disease, with clinical trials exploring its effects
-
Investigation in amyotrophic lateral sclerosis (ALS) patients, with favorable safety findings
-
Effects on protein aggregation and cellular stress responses relevant to multiple neurodegenerative disorders
Clinical Trials and Research Findings
Transplantation
Rapamycin has been extensively studied in transplant recipients, demonstrating efficacy in preventing organ rejection while potentially offering advantages over calcineurin inhibitors in terms of nephrotoxicity.
Cancer Studies
Multiple clinical studies have explored rapamycin's anticancer properties:
-
Demonstrated activity against renal cell carcinoma, leading to approval of the rapamycin derivative everolimus for this indication
-
Prevention of cancer in organ transplant patients receiving rapamycin compared to other immunosuppressants
-
Ongoing trials evaluating rapamycin for cancer prevention in high-risk populations
Aging Research
Notable clinical studies investigating rapamycin's effects on aging include:
Study | Country | Design | Intervention | Duration (days) | Participants | Age (years) | Results |
---|---|---|---|---|---|---|---|
Mannick et al. (2014) | New Zealand and Australia | RCT | RAD001 | 70 | 218 | ~71 | Improved immune function in elderly |
Kraig et al. (2018) | USA | RCT phase 1 | Rapamycin | 120 | 8 | 81-95 | Demonstrated safety and tolerability |
Kraig et al. (2018) | USA | RCT phase 2 | Rapamycin | 60 | 17 | 70-95 | Favorable safety profile |
Mannick et al. (2021) | USA | RCT phase 2b | RTB101 | 168 | 473 | ~73 | Evaluation in patients with chronic diseases |
Table 2: Key clinical trials investigating rapamycin and its derivatives in aging
Neurodegenerative Disease Research
Recent clinical investigations in neurodegenerative conditions:
Study | Disease | Design | Findings |
---|---|---|---|
Nussenblatt et al. (2010) | Age-related macular degeneration | RCT phase 1/2 | Evaluation in patients with choroidal neovascularization |
Petrou et al. (2014) | Geographic atrophy | RCT | Assessment in elderly patients with retinal degeneration |
Palma et al. (2022) | Multiple system atrophy | RCT | Safety and preliminary efficacy in patients with parkinsonian or cerebellar predominant symptoms |
Alzheimer's Disease study (2025) | Early-stage Alzheimer's | Open-label pilot | Evaluation of weekly rapamycin pharmacokinetics in patients with early-stage disease |
Table 3: Clinical studies of rapamycin in neurodegenerative conditions
Molecular Effects on Cellular Processes
Effects on Cell Proliferation and Senescence
Rapamycin exhibits distinct effects on cellular aging processes:
-
Decelerates cell proliferation while preserving proliferative potential
-
Suppresses both cell growth and geroconversion (transition from quiescence to senescence)
-
Reduces cellular hyperfunction, which underlies many age-related pathologies according to the hyperfunction theory of aging
-
Affects cell cycle progression by causing G1 arrest through inhibition of cdk2-cyclin E complex activity
Autophagy Regulation
Rapamycin is a potent inducer of autophagy:
-
Inhibition of mTORC1 activates autophagy by affecting Unc-51-like autophagy-activating kinases
-
Enhanced autophagy promotes clearance of protein aggregates and damaged organelles
-
This may contribute to rapamycin's neuroprotective effects in neurodegenerative disorders characterized by protein aggregation
Immunomodulatory Effects
The immunosuppressive effects of rapamycin involve:
-
Inhibition of T and B cell activation in response to interleukin-2
-
Prevention of the decline of p27 cdk inhibitor that normally follows IL-2 stimulation
-
Effects on regulatory T cells, which may contribute to anti-inflammatory properties
-
Modification of monocyte and B cell populations, with potential implications for inflammation and autoimmunity
Limitations and Side Effects
Common Adverse Effects
Clinical use of rapamycin is associated with various side effects:
-
Increased susceptibility to infections due to immunosuppression
-
Hyperlipidemia (elevated cholesterol and triglycerides)
-
Thrombocytopenia and leukopenia
-
Delayed wound healing
-
Mouth ulcers and stomatitis
-
Peripheral edema
-
Proteinuria and potential kidney effects
Pharmacokinetic Limitations
Challenges in rapamycin administration include:
-
Variable bioavailability requiring therapeutic drug monitoring
-
Extensive metabolism by CYP3A4, leading to numerous drug interactions
-
Limited blood-brain barrier penetration, potentially reducing efficacy in neurological conditions
-
Narrow therapeutic window, particularly for immunosuppressive applications
Regulatory and Clinical Considerations
Important considerations for clinical use:
-
Need for careful patient selection and monitoring
-
Potential requirement for dose adjustments based on therapeutic drug monitoring
-
Limitations in certain populations, such as pregnant women and children
-
Balancing efficacy and side effects, particularly for non-transplant indications
Future Directions
Novel Formulations and Derivatives
Ongoing research focuses on:
-
Development of rapamycin analogs (rapalogs) with improved pharmacokinetic properties
-
Formulations with enhanced tissue specificity or blood-brain barrier penetration
-
Combination therapies to enhance efficacy while minimizing side effects
-
Optimization of intermittent dosing regimens to maximize benefits while reducing adverse effects
Expanding Clinical Applications
Promising areas of future investigation include:
-
Age-related conditions beyond those currently under investigation
-
Neurodegenerative disorders, with ongoing trials in Alzheimer's disease and other conditions
-
Metabolic disorders and diabetes
Aging Biology and Geroscience
Rapamycin continues to be a key tool in understanding aging mechanisms:
-
Investigation of rapamycin's effects on the hallmarks of aging
-
Use as a probe to understand mTOR's role in aging and age-related diseases
-
Development of biomarkers to assess rapamycin's effects on biological aging
-
Integration into broader geroscience approaches to target aging processes
Mass Molarity Calculator
- mass of a compound required to prepare a solution of known volume and concentration
- volume of solution required to dissolve a compound of known mass to a desired concentration
- concentration of a solution resulting from a known mass of compound in a specific volume