L-Homoserine is a metabolite found in or produced by Escherichia coli (strain K12, MG1655).
L-homoserine is a natural product found in Glycine max, Capsicum annuum, and other organisms with data available.
L-homoserine
CAS No.: 672-15-1
VCID: VC21539474
Molecular Formula: C4H9NO3
Molecular Weight: 119.12 g/mol
* For research use only. Not for human or veterinary use.
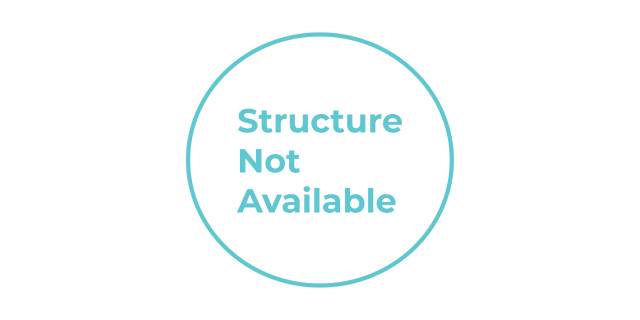
Description |
L-Homoserine is a non-proteinogenic amino acid, meaning it is not one of the standard amino acids encoded by DNA for protein synthesis. It plays a crucial role in the biosynthesis of essential amino acids such as methionine, threonine, and isoleucine. Structurally, L-homoserine is similar to serine but with an additional methylene group in its side chain, making it a more reactive variant . Biosynthesis and Metabolic RoleL-Homoserine is synthesized through the reduction of aspartic acid via aspartate semialdehyde. This process is crucial for the biosynthesis of methionine, threonine, and isoleucine, which are essential amino acids necessary for various biological functions . The synthesis of L-homoserine involves homoserine dehydrogenase, an enzyme that catalyzes the conversion of aspartate semialdehyde to homoserine . Uses and ApplicationsL-Homoserine serves as an internal standard for the analysis of neurotransmitters and amino acids. Its role in biosynthesis makes it a valuable compound in understanding metabolic pathways and in the production of essential amino acids . Additionally, derivatives of homoserine lactones have been explored for their potential to disrupt bacterial signaling, making pathogens more susceptible to antibiotics . Research Findings and ImplicationsRecent studies have highlighted the importance of understanding the metabolic pathways involving L-homoserine. For instance, its role in the biosynthesis of methionine and threonine underscores its significance in nutritional and biochemical research. Moreover, the exploration of homoserine lactones in bacterial signaling has opened new avenues for developing novel antimicrobial strategies . |
---|---|
CAS No. | 672-15-1 |
Product Name | L-homoserine |
Molecular Formula | C4H9NO3 |
Molecular Weight | 119.12 g/mol |
IUPAC Name | (2S)-2-amino-4-hydroxybutanoic acid |
Standard InChI | InChI=1S/C4H9NO3/c5-3(1-2-6)4(7)8/h3,6H,1-2,5H2,(H,7,8)/t3-/m0/s1 |
Standard InChIKey | UKAUYVFTDYCKQA-VKHMYHEASA-N |
Isomeric SMILES | C(CO)[C@@H](C(=O)O)N |
SMILES | C(CO)C(C(=O)O)N |
Canonical SMILES | C(CO)C(C(=O)O)N |
Melting Point | 203 °C |
Physical Description | Solid |
Solubility | 1000.0 mg/mL |
Synonyms | L-homoserine;672-15-1;(s)-2-amino-4-hydroxybutanoicacid;(s)-homoserine;(S)-2-Amino-4-hydroxybutyricacid;(2S)-2-amino-4-hydroxybutanoicacid;h-hoser-oh;Homoserine(VAN);2-Amino-4-hydroxybutyricacid;2-amino-4-hydroxybutyrate;UNII-6KA95X0IVO;2-amino-4-hydroxy-Butyrate;2-amino-4-hydroxy-L-Butyrate;2-amino-4-hydroxy-Butyricacid;CHEBI:15699;(S)-2-Amino-4-hydroxybutanoate;2-amino-4-hydroxybutanoate;UKAUYVFTDYCKQA-VKHMYHEASA-N;(S)-2-amino-4-hydroxy-Butanoate;2-amino-4-hydroxy-L-Butyricacid;MFCD00063090;(S)-2-amino-4-hydroxy-Butanoicacid;HSE;(2S)-2-amino-4-hydroxy-butanoicacid;Butanoicacid,2-amino-4-hydroxy-,(S)- |
Reference | Bastard et al. Parallel evolution of non-homologous isofunctional enzymes in methionine biosynthesis. Nature Chemical Biology, doi: 10.1038/nchembio.2397, published online 5 June 2017 |
PubChem Compound | 12647 |
Last Modified | Aug 15 2023 |
Mass Molarity Calculator
- mass of a compound required to prepare a solution of known volume and concentration
- volume of solution required to dissolve a compound of known mass to a desired concentration
- concentration of a solution resulting from a known mass of compound in a specific volume