L-homoserine lactone is the L-enantiomer of homoserine lactone. It is a conjugate base of a L-homoserine lactone(1+). It is an enantiomer of a D-homoserine lactone.
L-homoserine lactone
CAS No.: 2185-02-6
Cat. No.: VC21539281
Molecular Formula: C4H7NO2
Molecular Weight: 101,11*36,45 g/mole
* For research use only. Not for human or veterinary use.
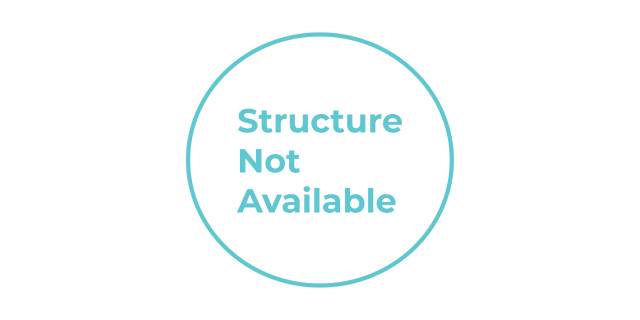
CAS No. | 2185-02-6 |
---|---|
Molecular Formula | C4H7NO2 |
Molecular Weight | 101,11*36,45 g/mole |
IUPAC Name | (3S)-3-aminooxolan-2-one |
Standard InChI | InChI=1S/C4H7NO2/c5-3-1-2-7-4(3)6/h3H,1-2,5H2/t3-/m0/s1 |
Standard InChI Key | QJPWUUJVYOJNMH-VKHMYHEASA-N |
Isomeric SMILES | C1COC(=O)[C@H]1N |
SMILES | C1COC(=O)C1N |
Canonical SMILES | C1COC(=O)C1N |
Chemical Structure and Properties
Molecular Structure and Identification
L-homoserine lactone (C4H7NO2) is the L-enantiomer of homoserine lactone with a molecular weight of 101.10 g/mol. It is systematically named as (3S)-3-aminooxolan-2-one or (S)-3-aminodihydrofuran-2(3H)-one . The compound features a five-membered lactone ring (cyclic ester) with an amino group at the 3-position. Its CAS number is 2185-02-6, and it is identified as a conjugate base of L-homoserine lactone(1+) . The structure includes a five-membered lactone ring, an amino group at the C-3 position, and S-stereochemistry at the C-3 position.
Physical and Chemical Properties
L-homoserine lactone has distinct chemical properties that influence its biological behavior. The lactone ring is susceptible to hydrolysis under aqueous conditions, with a typical half-life of approximately 12-48 hours at pH 7 . This hydrolysis results in the opening of the ring structure, leading to the formation of homoserine, which is biologically inactive in bacterial quorum sensing systems but may have other significant biological effects, particularly in plants .
The hydrochloride salt form of L-homoserine lactone (CAS: 2185-03-7) demonstrates good solubility in both DMSO and PBS buffer (pH 7.2) at concentrations up to 10 mg/ml, making it suitable for various laboratory applications . For research applications, proper storage at -20°C is recommended, with different stability periods depending on storage conditions: up to 6 months at -80°C and approximately 1 month at -20°C .
Stereochemistry and Biological Relevance
The stereochemistry of L-homoserine lactone is crucial for its biological activity. The L-enantiomer (S-configuration) is functionally distinct from its D-enantiomer counterpart in terms of biological recognition and function . Research demonstrates that the stereochemical configuration significantly impacts biological responses in both bacterial and plant systems, with markedly different responses observed between L-homoserine lactone and D-homoserine lactone .
Synthesis and Production
Chemical Synthesis Methods
Multiple synthetic routes have been developed for the production of L-homoserine lactone and its derivatives. One common approach involves the preparation of N-acyl-L-homoserine lactones (AHLs) using Schotten-Baumann conditions, where L-homoserine lactone hydrobromide is coupled with various acid chlorides . This method yields AHLs with excellent enantiomeric excess, indicating the absence of racemization during the coupling process .
A robust linear route has been established for the synthesis of β-ketoamide AHLs, including N-(3-oxooctanoyl-L-homoserine lactone (OOHL), N-(3-oxodecanoyl)-L-homoserine lactone (ODHL), and N-(3-oxododecanoyl)-L-homoserine lactone (OdDHL) . The yields from these synthetic approaches are typically high, ranging from 86% to 98%, as shown in Table 1.
Table 1: AHLs synthesized using the Schotten-Baumann coupling procedure
Compound | n (carbon chain length) | Yield (%) | Enantiomeric excess (%) |
---|---|---|---|
N-butanoyl-L-homoserine lactone (BHL) | 2 | 86 | >99 |
N-hexanoyl-L-homoserine lactone (HHL) | 4 | 97 | >99 |
N-octanoyl-L-homoserine lactone (OHL) | 6 | 96 | >99 |
N-decanoyl-L-homoserine lactone (DHL) | 8 | 98 | >99 |
N-dodecanoyl-L-homoserine lactone (dDHL) | 10 | 96 | >99 |
More recently, researchers have developed modified N-acyl-L-homoserine lactone compounds by adding tert-butoxycarbonyl (Boc) groups in different positions, resulting in compounds such as N-dodecanoyl-L-homoserine lactone-3-t-butoxycarbonyl hydrazone and N-(t-butoxycarbonyl)-3-((t-butoxycarbonyl)oxy)-dodecanoyl-L-homoserine lactone .
Biological Production
In biological systems, L-homoserine lactone is a key component of AHLs, which are synthesized by LuxI-type synthases in many Gram-negative bacteria . For example, in Vibrio fischeri, LuxI synthesizes N-(3-oxo-C6)-L-homoserine lactone . Different bacterial species produce varying AHLs with different acyl chain lengths (ranging from C4 to C18), degrees of saturation, or modifications at the third carbon of the acyl chain .
In Pseudomonas aeruginosa, the LasI enzyme directs the synthesis of N-(3-oxododecanoyl)-L-homoserine lactone (OdDHL), which activates the transcriptional regulator LasR . Similarly, the VsmI enzyme in P. aeruginosa produces N-butanoyl-L-homoserine lactone (BHL) and N-hexanoyl-L-homoserine lactone (HHL) in a molar ratio of approximately 15:1 .
Biological Significance
Role in Bacterial Quorum Sensing
L-homoserine lactone serves as a core component of AHLs, which function as dedicated cell-to-cell signaling molecules in many species of the class Proteobacteria . These signaling molecules are central to bacterial quorum sensing, a communication system that allows bacteria to monitor population density and coordinate gene expression accordingly .
The AHL-mediated quorum sensing systems regulate numerous important biological functions, including:
-
Bioluminescence
-
Biofilm formation
-
Virulence factor production
-
Secondary metabolite production
-
Toxicity
-
Enzymatic tissue degradation
-
Flagellum-dependent motility
-
Adhesion
These signaling systems are particularly important in many pathogenic bacteria, including Pseudomonas aeruginosa, Burkholderia cenocepacia, and Erwinia carotovora, where they regulate virulence factor production and biofilm formation .
Structure-Activity Relationships
The structure of AHLs, particularly the nature of the acyl side chain, determines the specificity of the quorum sensing system . Various AHL structures have been identified, with signal specificity depending on the length and substitutions in the acyl side chain . These molecules typically reach concentrations of around 10 μM in laboratory cultures of quorum-sensing bacteria .
Research has shown that the lactone ring is essential for AHLs to bind to LuxR-type receptors in bacteria, while the acyl group influences the specificity and potency of the interaction . This structure-activity relationship has been leveraged in the design of synthetic AHL analogs with potential applications as quorum sensing inhibitors .
Interaction with Receptor Proteins
AHLs interact with LuxR-type receptors to modulate gene expression through a well-defined molecular mechanism . After binding to the AHL signal molecule, the LuxR homologue is activated or inhibited as a transcription factor, which in turn regulates the expression of target genes .
The crystal structure of the AHL-lactonase from Bacillus thuringiensis complexed with L-homoserine lactone has provided valuable insights into the molecular interactions involved in AHL recognition and degradation . This structure reveals that the lactone ring and carbonyl oxygens of homoserine lactone interact with zinc ions in the enzyme's active site, with additional hydrogen bonding interactions involving specific amino acid residues .
Metabolism and Degradation
Enzymatic Degradation
Several organisms have developed mechanisms to degrade AHLs, potentially as a strategy to disrupt bacterial communication. Two main enzymatic pathways for AHL degradation have been identified:
-
Lactonases: These enzymes hydrolyze the lactone ring of AHLs, rendering them inactive in quorum sensing . The AHL-lactonase from Bacillus thuringiensis shows remarkable structural similarities to glyoxalase II and RNase Z proteins, members of the metallo-β-lactamase superfamily . This enzyme contains two zinc ions involved in catalysis, with a proposed mechanism involving a nucleophilic attack by a water/hydroxide bridging the two Zn2+ ions on the substrate's carbonyl carbon .
-
Amidases/Aminoacylases: These enzymes cleave the amide bond between the acyl chain and the homoserine lactone . For instance, Variovorax paradoxus can utilize AHLs as both energy and nitrogen sources, with the first step in metabolism involving a carboxypeptidase or aminoacylase that releases the fatty acid from the homoserine lactone (HSL) .
The detailed catalytic mechanism of AHL-lactonase has been elucidated through structural and functional studies. The reaction follows a conserved mechanism involving:
-
Nucleophilic attack by a water/hydroxide bridging two zinc ions on the substrate's carbonyl carbon
-
Enhanced polarization of the carbonyl bond due to interactions with the zinc ions
-
Formation of a negatively charged intermediate stabilized by interactions with zinc
-
Protonation of the leaving group by a tyrosine residue acting as a general acid
Environmental Fate
The degradation of AHLs in the environment occurs through both abiotic and biotic processes . Abiotic factors, such as pH and temperature, influence the rate of lactone ring hydrolysis . In biological contexts, certain bacteria like Variovorax paradoxus can metabolize AHLs completely, using them as sources of energy and nitrogen .
When Variovorax paradoxus grows on AHLs, homoserine lactone accumulates in the culture medium, suggesting that acyl-peptide bond cleavage occurs either outside the cells or through an export system for intracellular HSL . Further metabolism of HSL involves cleavage of the ring, with partial mineralization of the carbon .
Experiments using radiolabeled substrates have shown that when Variovorax paradoxus is grown on C4-HSL with ammonium chloride as a nitrogen source, nearly half of the radiolabel is recovered as CO2, demonstrating significant cleavage of the homoserine lactone ring . Additionally, homoserine lactone has been identified as an accumulating intermediate in the degradation of AHLs by Variovorax paradoxus .
Applications and Research
Research Tools
L-homoserine lactone and its derivatives serve as valuable research tools for studying bacterial communication and quorum sensing . Synthetic AHLs and analogs allow researchers to investigate the specificity and sensitivity of quorum sensing systems across different bacterial species .
Recent research has utilized L-homoserine lactone in developing biosensor-based systems for detecting and quantifying AHLs in bacterial cultures . These biosensors employ various detection methods, including fluorescence- and colorimetric-based approaches, with different detection thresholds for various AHLs .
Gas chromatography-tandem mass spectrometry (GC-MS) has been employed for more reliable and comprehensive detection and identification of AHLs and related compounds, including L-homoserine lactone . Such analytical techniques are crucial for confirming the presence and identity of these signaling molecules in complex biological samples .
Pharmaceutical Applications
The understanding of L-homoserine lactone in bacterial quorum sensing has led to interest in developing antiinfection therapies that target these communication systems . AHL-lactonases, which hydrolyze AHLs into inactive products, are particularly promising for blocking quorum-sensing systems without directly killing bacteria, potentially reducing the selective pressure for antibiotic resistance .
Modified AHLs, such as those with added tert-butoxycarbonyl (Boc) groups in different positions, are being investigated for their potential to interfere with bacterial quorum sensing, particularly in pathogenic bacteria like Pseudomonas aeruginosa . These modified compounds represent a novel approach to anti-virulence strategies that could complement traditional antibiotic treatments .
Agricultural Applications
Given the role of AHLs in plant-bacterial interactions, there is growing interest in utilizing L-homoserine lactone and related compounds for agricultural applications . Understanding how plants respond to these bacterial signaling molecules could lead to novel approaches for crop protection and enhancement .
L-homoserine itself attracts interest as a functional amino acid and potential precursor for the synthesis of various compounds, including O-acetylhomoserine and 2,4-dihydroxybutyrate . Recent metabolic engineering efforts have achieved efficient production of L-homoserine from glucose in Escherichia coli, with reported yields of up to 110 g/L homoserine with a yield of 0.64 g/g glucose and productivity of 1.82 g/L/h .
Plant Responses to L-Homoserine Lactone
Molecular Mechanisms
The molecular mechanisms underlying plant responses to L-homoserine lactone and related compounds are still being elucidated . Evidence suggests that fatty acid amide hydrolase (FAAH) may play a role in the plant perception of AHLs . Studies with FAAH-deficient Arabidopsis thaliana mutants (ΔAtFAAH) showed insensitivity to OdDHL but retained a biphasic response to L-homoserine, suggesting that AHL cleavage by FAAH might be involved in the plant's response to these bacterial signaling molecules .
This finding indicates that plants may recognize and respond to both intact AHLs and their degradation products through distinct mechanisms . The stereochemical specificity of these responses suggests the involvement of specific receptor proteins or enzymes that can discriminate between L- and D-enantiomers .
Future Perspectives and Challenges
The study of L-homoserine lactone continues to evolve, with several promising directions for future research:
-
Development of quorum sensing inhibitors based on L-homoserine lactone for novel antimicrobial strategies
-
Exploration of the ecological significance of AHL degradation in natural environments
-
Investigation of the potential agricultural applications of L-homoserine lactone and its derivatives
-
Further characterization of the plant perception mechanisms for AHLs and their degradation products
-
Engineering of synthetic signaling systems based on L-homoserine lactone for biotechnological applications
Challenges in this field include the complexity of bacterial communication networks, the potential for off-target effects when manipulating quorum sensing systems, and the need for improved methods to detect and quantify L-homoserine lactone and related compounds in complex biological matrices .
Mass Molarity Calculator
- mass of a compound required to prepare a solution of known volume and concentration
- volume of solution required to dissolve a compound of known mass to a desired concentration
- concentration of a solution resulting from a known mass of compound in a specific volume