L-Threoninol
CAS No.: 3228-51-1
Cat. No.: VC21538221
Molecular Formula: C4H11NO2
Molecular Weight: 105.14 g/mol
* For research use only. Not for human or veterinary use.
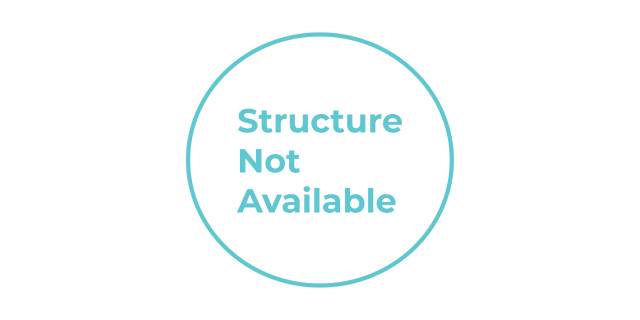
CAS No. | 3228-51-1 |
---|---|
Molecular Formula | C4H11NO2 |
Molecular Weight | 105.14 g/mol |
IUPAC Name | (2R,3R)-2-aminobutane-1,3-diol |
Standard InChI | InChI=1S/C4H11NO2/c1-3(7)4(5)2-6/h3-4,6-7H,2,5H2,1H3/t3-,4-/m1/s1 |
Standard InChI Key | MUVQIIBPDFTEKM-QWWZWVQMSA-N |
Isomeric SMILES | C[C@H]([C@@H](CO)N)O |
SMILES | CC(C(CO)N)O |
Canonical SMILES | CC(C(CO)N)O |
Chemical Structure and Properties
L-Threoninol features an amino group and two hydroxyl groups positioned on a short carbon chain. The molecule maintains the L-configuration inherited from L-threonine, which proves crucial for its biological interactions and applications in stereochemically controlled syntheses. This stereochemistry distinguishes it from its D-isomer counterpart, as the spatial arrangement of functional groups significantly affects its molecular recognition properties and interactions with biological macromolecules .
The chemical formula of L-threoninol is C₄H₁₁NO₂, representing its basic structure before any modifications. When incorporated into more complex structures, the primary hydroxyl group often serves as a point of attachment for nucleobases in artificial nucleic acid synthesis, while the secondary hydroxyl group can be protected or modified to control the conformational flexibility of the resulting molecules .
One particularly noteworthy property of L-threoninol is its optimal structural rigidity when compared to other acyclic scaffolds used in artificial nucleic acid design. Research has demonstrated that L-threoninol-based nucleic acid analogs exhibit better hybridization properties with natural DNA and RNA than their D-threoninol counterparts or other flexible scaffolds like serinol-based nucleic acids .
Synthesis Methods of L-Threoninol and Derivatives
General Synthesis Approach
The synthesis of L-threoninol typically begins with L-threonine as the starting material. The carboxylic acid group of L-threonine is reduced to obtain the primary alcohol while preserving the stereochemistry. Various reducing agents can be employed for this transformation, with sodium borohydride being commonly used in laboratory settings .
A general synthetic route involves:
-
Protection of the amino group of L-threonine
-
Activation of the carboxylic acid group
-
Reduction of the activated carboxylic acid derivative
-
Deprotection of the amino group
Synthesis of Protected L-Threoninol Derivatives
For applications in nucleic acid chemistry and pharmaceutical development, L-threoninol is often used in protected forms. One such derivative is Fmoc-O-tert-butyl-L-threoninol, which has been synthesized through a multi-step process as detailed in patent literature .
The synthesis method described in the patent involves:
-
Conversion of L-threonine to its methyl ester hydrochloride by treatment with thionyl chloride in methanol at low temperature (below 0°C) followed by reflux
-
Protection of the amino group using appropriate protecting groups
-
Protection of the secondary hydroxyl group with a tert-butyl group
-
Reduction of the ester to primary alcohol using sodium borohydride
-
Introduction of the Fmoc protecting group on the amino function
This synthetic approach addresses previous issues of high risk, high cost, and low yield, providing an improved method for obtaining protected L-threoninol derivatives with higher efficiency .
Synthesis of O-Benzyl-L-threoninol
O-Benzyl-L-threoninol represents another important derivative of L-threoninol, where the secondary hydroxyl group is protected as a benzyl ether. This modification provides stability during various chemical transformations while allowing for selective functionalization of the remaining functional groups. The benzyl protecting group can be removed under mild conditions when needed, making this derivative particularly useful in multi-step synthetic sequences .
Applications in Biochemical Research
Acyclic Nucleic Acid Analogs
One of the most significant applications of L-threoninol is in the development of artificial nucleic acids. The acyclic L-threoninol nucleic acid (L-aTNA) has been studied for its ability to form stable duplexes with natural DNA and RNA. Research has shown that L-aTNA possesses suitable structural rigidity that enables effective cross-pairing with both DNA and RNA molecules .
Compared to other artificial nucleic acid scaffolds, L-aTNA demonstrates superior hybridization properties. Studies have indicated that L-aTNA forms more stable duplexes with DNA and RNA than D-aTNA (based on D-threoninol) or serinol nucleic acid (SNA). This enhanced stability is attributed to the optimal rigidity of the L-form, which promotes more favorable interactions with natural nucleic acids .
The unique properties of L-aTNA make it valuable for applications such as:
-
Antisense oligonucleotides for gene silencing
-
Diagnostic probes for nucleic acid detection
-
Research tools for studying nucleic acid interactions
-
Potential therapeutic agents targeting specific nucleic acid sequences
Pharmaceutical Applications
L-Threoninol and its derivatives serve as valuable intermediates in pharmaceutical synthesis. O-Benzyl-L-threoninol, for instance, is utilized in the development of drugs targeting neurological disorders. The compound's stereochemically defined structure makes it an ideal building block for synthesizing complex pharmaceutical compounds where stereochemical control is crucial .
The applications of L-threoninol derivatives in pharmaceutical development include:
-
Synthesis of chiral drug candidates
-
Development of neurological disorder treatments
-
Creation of peptide-based therapeutics
-
Synthesis of bioactive compounds with defined stereochemistry
Biochemical Studies
L-Threoninol derivatives are employed in biochemical research to study enzyme activity and protein interactions. These compounds can serve as probes or substrates in enzymatic assays, helping researchers understand complex biological processes at the molecular level .
Additionally, research on related compounds like L-threonine has shown interesting biological effects such as promoting healthspan in model organisms like Caenorhabditis elegans. While these findings specifically relate to L-threonine rather than L-threoninol, they highlight the biological significance of this class of compounds and suggest potential directions for future research on L-threoninol derivatives .
Comparative Analysis with Other Acyclic Scaffolds
L-Threoninol-based nucleic acids offer distinct advantages over other acyclic scaffolds used in artificial nucleic acid design. The table below summarizes the comparative properties of different acyclic nucleic acid analogs:
Property | L-aTNA (L-Threoninol-based) | D-aTNA (D-Threoninol-based) | SNA (Serinol-based) |
---|---|---|---|
Duplex stability with DNA | Higher | Lower | Lower |
Duplex stability with RNA | Higher | Lower | Lower |
Structural rigidity | Optimal | Less optimal | Less optimal |
Nuclease resistance | High | High | High |
Synthetic accessibility | Moderate | Moderate | High |
This comparative analysis demonstrates that L-threoninol provides an ideal balance of structural flexibility and rigidity that promotes effective interactions with natural nucleic acids while maintaining the beneficial properties of acyclic scaffolds, such as resistance to nuclease degradation .
Recent Research Findings
Recent studies have explored the molecular basis for the superior hybridization properties of L-threoninol-based nucleic acids. The research indicates that the stereochemical configuration of L-threoninol imposes conformational constraints that favor the adoption of structures compatible with the helical geometries of natural nucleic acids. This structural complementarity enhances base stacking and hydrogen bonding interactions, resulting in more stable duplexes .
Furthermore, the acyclic nature of L-threoninol-based nucleic acids provides advantages in terms of nuclease resistance. Unlike natural nucleic acids with ribose or deoxyribose rings, the significant structural differences in acyclic scaffolds make them poor substrates for nucleases, enhancing their stability in biological environments. This property is particularly valuable for applications requiring nucleic acid stability in cellular contexts .
Industrial Applications
Beyond research applications, L-threoninol derivatives have found uses in various industrial sectors:
Cosmetic Industry
O-Benzyl-L-threoninol has been incorporated into skincare formulations for its moisturizing properties. The compound contributes to enhancing the texture and effectiveness of creams and lotions, demonstrating the versatility of L-threoninol derivatives beyond biochemical research .
Food Industry
In the food sector, certain L-threoninol derivatives serve as flavoring agents or additives. These compounds can improve the sensory attributes of food products while maintaining safety and quality standards .
Analytical Chemistry
L-Threoninol derivatives are employed in various analytical techniques, aiding in the detection and quantification of other substances in complex mixtures. Their defined stereochemistry and functional group arrangement make them valuable tools for developing selective analytical methods .
Mass Molarity Calculator
- mass of a compound required to prepare a solution of known volume and concentration
- volume of solution required to dissolve a compound of known mass to a desired concentration
- concentration of a solution resulting from a known mass of compound in a specific volume