Dihydrorobinetin
CAS No.: 4382-33-6
Cat. No.: VC21345415
Molecular Formula: C15H12O7
Molecular Weight: 304.25 g/mol
* For research use only. Not for human or veterinary use.
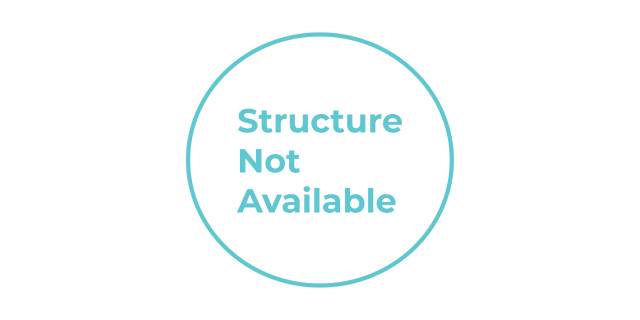
CAS No. | 4382-33-6 |
---|---|
Molecular Formula | C15H12O7 |
Molecular Weight | 304.25 g/mol |
IUPAC Name | 3,7-dihydroxy-2-(3,4,5-trihydroxyphenyl)-2,3-dihydrochromen-4-one |
Standard InChI | InChI=1S/C15H12O7/c16-7-1-2-8-11(5-7)22-15(14(21)12(8)19)6-3-9(17)13(20)10(18)4-6/h1-5,14-18,20-21H |
Standard InChI Key | VSJCDPYIMBSOKN-UHFFFAOYSA-N |
Isomeric SMILES | C1=CC2=C(C=C1O)O[C@@H]([C@H](C2=O)O)C3=CC(=C(C(=C3)O)O)O |
SMILES | C1=CC2=C(C=C1O)OC(C(C2=O)O)C3=CC(=C(C(=C3)O)O)O |
Canonical SMILES | C1=CC2=C(C=C1O)OC(C(C2=O)O)C3=CC(=C(C(=C3)O)O)O |
Chemical Identity and Structure
Molecular Characteristics
Dihydrorobinetin is chemically identified as (2R-trans)-2,3-dihydro-3,7-dihydroxy-2-(3,4,5-trihydroxyphenyl)-4-benzopyrone with the molecular formula C15H12O7 and a molecular weight of 304.25 g/mol . This compound belongs to the flavanone subclass of flavonoids, specifically categorized as a dihydroflavonol with five hydroxyl groups strategically positioned across its structure . The compound is also known by several synonyms including 2,3-Dihydrorobinetin, Robinetin dihydro-, and (2R,3R)-3,7-Dihydroxy-2-(3,4,5-trihydroxyphenyl)chroman-4-one . The stereochemistry at positions 2 and 3 is established as 2R,3R (trans), which is important for its biological activity and molecular recognition properties .
Structural Features
The chemical structure of dihydrorobinetin features a basic flavanone skeleton with a 2,3-dihydro-4-benzopyrone core . This structure is characterized by hydroxyl groups at positions 3, 7, 3', 4', and 5', with the hydroxyl groups at positions 3', 4', and 5' located on the B-ring (3,4,5-trihydroxyphenyl group) . The presence of these multiple hydroxyl groups contributes significantly to the compound's antioxidant properties through their ability to donate hydrogen atoms to neutralize free radicals . The 2,3-dihydro configuration distinguishes dihydrorobinetin from its oxidized counterpart, robinetin, and influences its solubility, reactivity, and biological activity .
Natural Sources and Distribution
Plant Sources
Dihydrorobinetin has been identified in several plant species, with particularly notable concentrations in Robinia pseudoacacia L. (black locust) wood and Adenanthera pavonina . The heartwood of Robinia pseudoacacia is considered one of the richest natural sources of this compound and has been extensively studied for efficient extraction methods . Additionally, dihydrorobinetin has been reported as an active constituent in Pterocarpus marsupium (Indian Kino, locally known as "Asana"), a plant traditionally used in Ayurvedic medicine for treating diabetes mellitus . The distribution of dihydrorobinetin across these plant species suggests an evolutionary significance, possibly related to defense mechanisms or ecological adaptations.
Extraction and Purification Methods
Solvent Extraction Optimization
Research has demonstrated that the extraction efficiency of dihydrorobinetin from plant material is significantly influenced by the solvent system employed . In a comprehensive study by Destandau et al., various solvent systems were evaluated for their ability to extract dihydrorobinetin from Robinia pseudoacacia wood, with results indicating that binary mixtures of polar organic solvents with water yield superior results compared to single-component systems . Specifically, an ethanol/water mixture (80/20) showed high extraction efficiency with a dihydrorobinetin concentration of 870 mg·L⁻¹, while methanol/water (80/20) and acetone/water (80/20) yielded 820 mg·L⁻¹ and 780 mg·L⁻¹, respectively . Pure water proved significantly less effective, yielding only 240 mg·L⁻¹ of dihydrorobinetin, demonstrating the importance of solvent selection in extraction protocols .
Critical Extraction Parameters
The optimization of extraction conditions revealed several critical parameters that significantly influence dihydrorobinetin yield:
Parameter | Value | DHRob Concentration (mg·L⁻¹) |
---|---|---|
Solvent | H₂O | 240 |
MeOH/H₂O 80/20 | 820 | |
EtOH/H₂O 80/20 | 870 | |
Acetone/H₂O 80/20 | 780 | |
Raw material to solvent ratio | 1% | 120 |
5% | 770 | |
9% | 1380 | |
13% | 1782 | |
Extraction time | 1 h | 1050 |
2 h | 1500 | |
4 h | 2000 | |
6 h | 1950 | |
24 h | 2023 |
The solid-to-liquid ratio (raw material to solvent) demonstrated a particularly strong influence, with higher ratios (up to 13%) resulting in substantially increased dihydrorobinetin concentration (up to 1782 mg·L⁻¹) . Extraction time also proved crucial, with concentration increasing rapidly during the first 4 hours (reaching 2000 mg·L⁻¹) and then plateauing with minimal additional gains over extended periods up to 24 hours . Further optimization indicated that a 50/50 ethanol/water mixture provided the highest concentration of dihydrorobinetin at 2500 mg·L⁻¹, representing the optimal balance between solvent polarity and extraction efficiency .
Gram-Scale Purification via Centrifugal Partition Chromatography
The purification of dihydrorobinetin to high levels of purity has been successfully achieved using Centrifugal Partition Chromatography (CPC), a liquid-liquid partition technique particularly well-suited for the separation of natural products . The process begins with the concentration of crude extract, during which an interesting phase separation occurs—the evaporation of ethanol leads to the precipitation of less polar compounds (primarily robinetin), resulting in two distinct phases: a liquid aqueous brown phase (S phase) containing 38% of concentrated dihydrorobinetin and only 2% of robinetin, and a yellow solid phase (D phase) containing 5.5% of dihydrorobinetin and 34% of robinetin . This natural separation during the concentration step provides an initial fractionation that enhances subsequent purification efficiency.
CPC Purification Parameters
The CPC purification of dihydrorobinetin employs a carefully optimized biphasic solvent system composed of heptane/ethyl acetate/methanol/water in a ratio of 1:4:1:4, providing a partition coefficient (K) for dihydrorobinetin of 1.4 and for robinetin of 3.8 . This difference in partition coefficients enables effective separation of these structurally similar compounds. Using a 50 mL CPC column with a sample loading of 35 mg crude extract, researchers were able to recover 11.3 mg of purified dihydrorobinetin, demonstrating the scalability and efficiency of this technique for obtaining research-grade material . The capability to purify dihydrorobinetin at gram scale opens possibilities for both research applications and potential commercial development of products incorporating this bioactive compound.
Analytical Methods for Detection and Quantification
HPLC Analysis
High-Performance Liquid Chromatography (HPLC) serves as the primary analytical method for the detection and quantification of dihydrorobinetin in plant extracts and purified fractions . The optimized HPLC method employs a gradient elution system typically composed of 0.1% formic acid in water (phase A) and 0.1% formic acid in methanol/acetonitrile (50/50) (phase B) . UV detection at 280 nm provides optimal sensitivity for dihydrorobinetin based on its absorption maximum, though additional wavelengths (310 nm and 366 nm) may be monitored simultaneously to detect other flavonoids like robinetin that have different absorption maxima . Quantification is achieved through calibration curves established using standard solutions across a concentration range of 10-500 mg·L⁻¹, with dihydrorobinetin showing excellent linearity (R² = 0.9997) in the relationship between concentration and detector response .
Biological Activities
Antioxidant Properties
Dihydrorobinetin demonstrates exceptional antioxidant properties through multiple mechanisms, positioning it as a compound of significant interest for applications requiring free radical neutralization . The most notable antioxidant activity is its potent 1,1-diphenyl-2-picrylhydrazyl (DPPH) radical-scavenging capacity, with a half-maximal inhibitory concentration (IC₅₀) of just 1.3 μM, indicating extremely high efficiency in neutralizing this stable free radical . This potency likely derives from the compound's pentahydroxy structure, which provides multiple sites for hydrogen donation to quench free radicals. Additionally, dihydrorobinetin exhibits superoxide dismutase (SOD)-like activity with a half-maximal effective concentration of 27 μM, suggesting its ability to neutralize superoxide radicals that are implicated in oxidative stress and cellular damage .
Anti-Glycation Activity
Beyond its direct antioxidant effects, dihydrorobinetin demonstrates significant suppressive activity against the formation of advanced glycation end products (AGEs), with an IC₅₀ value of 132 μM . AGEs are harmful compounds formed when proteins or lipids become glycated as a result of exposure to reducing sugars, and their accumulation is associated with various age-related disorders and complications of diabetes mellitus. The inhibition of AGE formation represents an important protective mechanism against these pathological processes, suggesting potential applications for dihydrorobinetin in anti-aging and diabetes management strategies . This anti-glycation activity, combined with the compound's antioxidant properties, provides a multi-faceted approach to protecting against cellular damage and age-related deterioration.
Research Development and Future Directions
Future Research Directions
Despite the significant progress in understanding dihydrorobinetin, several research directions warrant further investigation to fully realize its potential . Detailed structure-activity relationship studies would help elucidate the precise molecular mechanisms underlying its various biological activities and potentially guide the development of synthetic analogues with enhanced properties. In vivo studies are needed to confirm the biological activities observed in vitro and to assess pharmacokinetic parameters such as absorption, distribution, metabolism, and excretion. Research into formulation strategies would address challenges related to stability and bioavailability, particularly important given the typical limitations in these areas for polyphenolic compounds. Additionally, clinical studies would be necessary to evaluate safety, efficacy, and optimal dosing for specific applications in human health and disease management.
Mass Molarity Calculator
- mass of a compound required to prepare a solution of known volume and concentration
- volume of solution required to dissolve a compound of known mass to a desired concentration
- concentration of a solution resulting from a known mass of compound in a specific volume