Isoquercitrin is under investigation in clinical trial NCT04622865 (Masitinib Combined With Isoquercetin and Best Supportive Care in Hospitalized Patients With Moderate and Severe COVID-19).
Hyperoside is a natural product found in Camellia sinensis, Geranium carolinianum, and other organisms with data available.
See also: Bilberry (part of); Menyanthes trifoliata leaf (part of); Crataegus monogyna flowering top (part of).
Hyperoside
CAS No.: 482-36-0
Cat. No.: VC21345155
Molecular Formula: C21H20O12
Molecular Weight: 464.4 g/mol
* For research use only. Not for human or veterinary use.
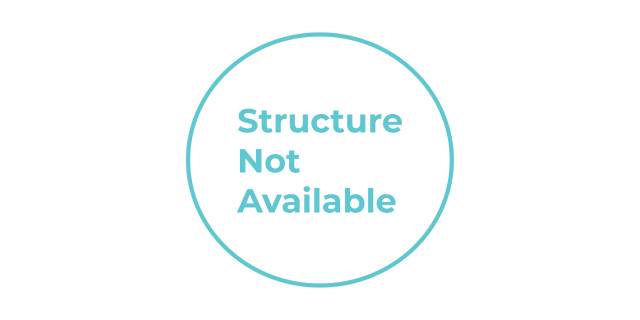
CAS No. | 482-36-0 |
---|---|
Molecular Formula | C21H20O12 |
Molecular Weight | 464.4 g/mol |
IUPAC Name | 2-(3,4-dihydroxyphenyl)-5,7-dihydroxy-3-[(2S,3R,4S,5R,6R)-3,4,5-trihydroxy-6-(hydroxymethyl)oxan-2-yl]oxychromen-4-one |
Standard InChI | InChI=1S/C21H20O12/c22-6-13-15(27)17(29)18(30)21(32-13)33-20-16(28)14-11(26)4-8(23)5-12(14)31-19(20)7-1-2-9(24)10(25)3-7/h1-5,13,15,17-18,21-27,29-30H,6H2/t13-,15+,17+,18-,21+/m1/s1 |
Standard InChI Key | OVSQVDMCBVZWGM-DTGCRPNFSA-N |
Isomeric SMILES | C1=CC(=C(C=C1C2=C(C(=O)C3=C(C=C(C=C3O2)O)O)O[C@H]4[C@@H]([C@H]([C@H]([C@H](O4)CO)O)O)O)O)O |
Canonical SMILES | C1=CC(=C(C=C1C2=C(C(=O)C3=C(C=C(C=C3O2)O)O)OC4C(C(C(C(O4)CO)O)O)O)O)O |
Melting Point | 232 - 233 °C |
Chemical Properties and Structure
Hyperoside, chemically known as quercetin 3-O-β-D-galactopyranoside, possesses a molecular formula of C21H20O12 with a molecular weight of 464.38 . This compound appears as yellowish needle-like crystals with a melting point ranging from 225-226°C . The substance exhibits specific optical rotation of -83° when dissolved in pyridine at a concentration of 0.2 . Regarding its solubility profile, hyperoside demonstrates limited solubility in common organic solvents; it is slightly soluble in dimethyl sulfoxide (DMSO) and methanol (especially when heated) . The compound has a predicted boiling point of 872.6±65.0°C and an estimated density of 1.87±0.1 g/cm³ .
Physical and Chemical Characteristics
Hyperoside is recognized for its stability under normal conditions and its characteristic reactions, including the formation of a cherry-red substance when treated with hydrochloric acid-magnesium powder . The compound produces a green coloration with ferric chloride and gives a positive result in the α-naphthol reaction . These distinctive chemical reactions serve as valuable tools for its identification and quality assessment in analytical procedures.
Structural Data
The following table summarizes the key physical and chemical properties of hyperoside:
Property | Value |
---|---|
CAS Number | 482-36-0 |
Molecular Formula | C21H20O12 |
Molecular Weight | 464.38 |
Melting Point | 225-226°C |
Boiling Point | 872.6±65.0°C (Predicted) |
Density | 1.87±0.1 g/cm³ (Predicted) |
Appearance | Light Yellow to Yellow Solid |
Solubility | DMSO (Slightly), Methanol (Slightly, Heated) |
pKa | 6.17±0.40 (Predicted) |
LogP | -0.111 (est) |
Natural Sources and Extraction Methods
Hyperoside occurs naturally in various plant species belonging to different families, including Hypericaceae, Rosaceae, Ericaceae, Leguminosae, and Celastraceae . It can be found in different plant organs such as flowers, leaves, and fruits . Historically, hyperoside was isolated from redosier dogwood by Nair et al. in 1960, with a content of approximately 0.075% .
Extraction Techniques
Several extraction methods have been developed to obtain hyperoside from plant sources. One optimized extraction process involves using 77.6% ethanol at 11.0 times the amount of plant material, with two extraction cycles of 2.7 hours each . This method, determined through response surface methodology, optimizes the extraction rate of hyperoside by considering key variables such as ethanol concentration, material-to-liquid ratio, and extraction duration .
An alternative extraction approach utilizes 60% ethanol at 20 times the amount of plant material, with four extraction cycles at 90°C for 2 hours each . This method, developed through orthogonal experimental design, provides a simple, accurate, and reproducible technique for hyperoside extraction . These extraction methodologies are crucial for obtaining high-quality hyperoside for research and pharmaceutical applications.
Synthetic Methods
While hyperoside is primarily obtained through natural extraction, synthetic methods have also been developed. One such approach utilizes rutin as a starting material and involves several chemical transformations including benzylation, acid hydrolysis, glycoside condensation through phase transfer catalysis, followed by deacetylation and debenzylation processes . This synthetic pathway provides an alternative source of hyperoside, especially when natural sources may be limited or when specific structural modifications are desired.
Pharmacological Properties
Hyperoside exhibits a diverse range of pharmacological activities that highlight its therapeutic potential in various disease conditions. These properties stem from its ability to modulate multiple biological pathways and cellular processes.
Analgesic Effects
Hyperoside demonstrates significant local analgesic effects without inducing dependence, positioning it as a promising alternative to conventional pain medications . Its analgesic potency exceeds that of aspirin but is less than morphine, making it a potentially valuable new local analgesic agent . The pain-relieving properties of hyperoside may be associated with its anti-inflammatory effects, as suggested by recent research .
Cardiovascular and Cerebrovascular Protection
One of the most notable properties of hyperoside is its protective effect on the cardiovascular and cerebrovascular systems. The compound has shown significant protective activity against myocardial ischemia and reperfusion injury, as well as cerebral ischemia and reperfusion damage . These cardioprotective and neuroprotective effects position hyperoside as a potential therapeutic agent for conditions such as myocardial infarction and stroke. Furthermore, the compound's ability to influence vascular health makes it relevant for the management of high blood pressure and related cardiovascular conditions .
Anti-inflammatory Effects
Hyperoside possesses robust anti-inflammatory properties through multiple mechanisms. In experimental models, intraperitoneal administration of hyperoside at a dose of 20mg/kg daily for seven days significantly inhibited inflammatory processes in rats . The compound modulates inflammatory responses by reducing the release of pro-inflammatory factors through various signaling pathways, including the Toll-like Receptor 4 (TLR4) pathway induced by lipopolysaccharide (LPS), and the Wnt/β-catenin and Sonic Hedgehog signaling pathways .
At the molecular level, hyperoside inhibits the production of key pro-inflammatory mediators such as tumor necrosis factor-alpha (TNF-α), interleukin-6 (IL-6), and nitric oxide (NO) . Concurrently, it promotes the production of anti-inflammatory factors like interleukin-10 (IL-10), creating a balanced anti-inflammatory effect . This bidirectional regulatory capability enhances its potential as an anti-inflammatory therapeutic agent.
Antidepressant Activity
In vivo experiments have demonstrated hyperoside's significant efficacy in improving depressive-like behavior in mice subjected to chronic stress . In the Chronic Unpredictable Mild Stress (CUMS) model, mice treated with hyperoside showed reduced immobility times in behavioral tests such as the Forced Swim Test (FST) and Tail Suspension Test (TST), along with improved sucrose preference rates compared to untreated stressed mice . These results indicate hyperoside's ability to alleviate depression-like symptoms induced by chronic stress.
The molecular mechanisms underlying hyperoside's antidepressant effects involve the inhibition of inflammasome-related molecules (NLRP1, ASC, and caspase-1) in the hippocampus, as well as the suppression of pro-inflammatory cytokines such as IL-18, IL-6, TNF-α, and IL-1β . Additionally, hyperoside modulates the expression of stress-related CXCL1 and CXCR2 while upregulating brain-derived neurotrophic factor (BDNF), a protein closely associated with antidepressant effects .
Anticancer Mechanisms
Hyperoside has demonstrated significant anticancer activities against various types of cancer, including lung, colorectal, bladder, pancreatic, and hepatocellular carcinoma . Its anticancer effects operate through multiple mechanisms that collectively contribute to its potential as an anticancer agent.
Cell Proliferation Inhibition
Hyperoside effectively inhibits the proliferation of cancer cells through various pathways. In lung cancer cell line A549, hyperoside suppresses the expression of cyclin D1 (CCND1) and cyclin-dependent kinase 4/6 (CDK4/CDK6), which are crucial for the G1/S transition in the cell cycle . This mechanism leads to cell cycle arrest in the G1 phase, thereby inhibiting cancer cell proliferation .
Furthermore, hyperoside interferes with multiple signaling pathways essential for cell proliferation. It inhibits key pathways such as mitogen-activated protein kinase (MAPK), mammalian target of rapamycin (mTOR), and Protein Kinase B (PKB/Akt), while reducing the expression of epidermal growth factor receptor (EGFR) . Since EGFR overexpression often promotes cancer cell proliferation, hyperoside's ability to lower EGFR levels contributes significantly to its antiproliferative effects .
Apoptosis Induction
Hyperoside promotes apoptosis (programmed cell death) in cancer cells in a dose-dependent manner . This effect is particularly evident in lung cancer cells and involves multiple apoptotic pathways . One mechanism by which hyperoside induces apoptosis is by disrupting anti-apoptotic mechanisms through inhibition of Bcl-2 protein expression downstream of the Akt signaling pathway .
Flow cytometry analyses have shown that hyperoside treatment significantly elevates levels of activated caspase-3 and caspase-9 in cancer cells, confirming its pro-apoptotic effects . These caspases are key executioners in the apoptotic cascade, and their activation plays a crucial role in initiating and executing the apoptotic program.
Autophagy Modulation
Hyperoside significantly influences autophagy, a cellular process involved in the degradation and recycling of cellular components. In ovarian cancer cells, hyperoside treatment increases levels of autophagy marker LC3B-II and enhances autophagosome formation . This is accompanied by increased expression of autophagy-related proteins Beclin-1 and decreased expression of the autophagy substrate p62 protein, indicating enhanced autophagy flux .
Interestingly, the autophagy-modulating effects of hyperoside appear to be mediated through progesterone receptor membrane component 1 (PGRMC1), as evidenced by enhanced colocalization between LC3B and PGRMC1 following hyperoside treatment . The overexpression of PGRMC1 augments hyperoside-induced autophagy and apoptosis, whereas PGRMC1 knockdown inhibits these processes .
Synergistic Effects with Chemotherapy
Hyperoside demonstrates potential for combination therapy with conventional chemotherapeutic agents. In experiments with ovarian cancer cells, co-administration of hyperoside and cisplatin resulted in significantly decreased cell viability and increased apoptotic cell proportion compared to single-agent treatment . This suggests that hyperoside enhances the cytotoxicity of cisplatin through PGRMC1-dependent autophagy regulation, potentially allowing for lower doses of chemotherapeutic agents and reduced side effects .
Molecular Pathways and Targets
The diverse biological activities of hyperoside are mediated through its interactions with multiple molecular pathways and targets. Understanding these mechanisms provides insights into its therapeutic potential and guides the development of targeted interventions.
Growth Factor Signaling
Hyperoside significantly interferes with growth factor-mediated signaling, which is crucial for regulating cell growth and differentiation. Specifically, it inhibits signaling pathways activated by growth factors such as platelet-derived growth factor (PDGF), epidermal growth factor (EGF), insulin-like growth factor (IGF), and TNF-α . These growth factors play critical roles in promoting cancer cell proliferation by binding to specific receptors on the cell membrane .
Through Western blot analysis, hyperoside has been shown to significantly reduce the expression of EGFR, a receptor often overexpressed in cancer cells . This inhibition of EGFR expression contributes to hyperoside's antiproliferative effects on cancer cells and highlights its potential in targeting aberrant growth factor signaling in cancer therapy.
Inflammation and Immune Regulation
Hyperoside modulates inflammatory processes by influencing various signaling pathways involved in the inflammatory response. It inhibits the TLR4 signaling pathway induced by LPS, as well as the Wnt/β-catenin and Sonic Hedgehog signaling pathways . These pathways play crucial roles in regulating inflammation and immune responses.
At the molecular level, hyperoside reduces the expression of inflammasome-related molecules NLRP1, ASC, and caspase-1 in the hippocampus, while inhibiting the mRNA levels of pro-inflammatory cytokines such as IL-18, IL-6, TNF-α, and IL-1β . By targeting these key mediators of inflammation, hyperoside effectively modulates the inflammatory response and exerts its anti-inflammatory effects.
Cell Cycle Regulation
Hyperoside's anticancer effects are partly mediated through its influence on cell cycle regulation. In lung cancer cells, hyperoside suppresses the expression of cyclin D1 and cyclin-dependent kinase 4/6, which are essential for the G1/S transition in the cell cycle . This suppression leads to cell cycle arrest in the G1 phase, preventing cancer cells from progressing through the cell cycle and proliferating .
Therapeutic Applications
The diverse pharmacological properties of hyperoside suggest its potential applications in various therapeutic areas. From cancer treatment to neurodegenerative disorders and inflammatory diseases, hyperoside offers promising opportunities for the development of novel therapeutic strategies.
Cancer Treatment
Hyperoside has shown remarkable anticancer potential against various types of cancer, including lung cancer, colorectal cancer, bladder cancer, pancreatic cancer, and hepatocellular carcinoma . Its anticancer effects are mediated through multiple mechanisms, including inhibition of cell proliferation, induction of apoptosis, modulation of autophagy, and enhancement of chemotherapy sensitivity .
In ovarian cancer cells, hyperoside enhances the cytotoxicity of cisplatin through PGRMC1-dependent autophagy regulation, suggesting its potential as an adjuvant in combination therapy . This synergistic effect could potentially allow for lower doses of chemotherapeutic agents, reducing side effects while maintaining therapeutic efficacy.
Neurodegenerative and Psychiatric Disorders
Hyperoside's neuroprotective and antidepressant properties highlight its potential in treating neurodegenerative and psychiatric disorders. Its ability to protect against cerebral ischemia and reperfusion injury positions it as a candidate for neuroprotective interventions in conditions such as stroke .
In depression models, hyperoside has demonstrated significant efficacy in improving depressive-like behavior in mice subjected to chronic stress . Its antidepressant effects involve modulation of inflammasome-related molecules and pro-inflammatory cytokines in the hippocampus, as well as upregulation of BDNF . These mechanisms suggest hyperoside's potential as an alternative or adjunct treatment for depression, particularly in cases where inflammation contributes to the pathophysiology.
Inflammatory and Age-Related Diseases
The anti-inflammatory properties of hyperoside make it a promising candidate for treating inflammatory diseases. Its ability to reduce the release of inflammatory factors while promoting anti-inflammatory mediators creates a balanced anti-inflammatory effect that could be beneficial in conditions characterized by chronic inflammation .
In age-related contexts, hyperoside treatment has been shown to inhibit the expression of aging markers p53 and p21, while reducing levels of pro-inflammatory factors IL-1 and TGF-β . Additionally, hyperoside downregulates autophagy-related proteins LC3 and Beclin1, decreasing autophagosome accumulation and potentially protecting against autophagy hyperactivity in aging . These effects suggest hyperoside's potential in managing age-related diseases and promoting healthy aging.
Mass Molarity Calculator
- mass of a compound required to prepare a solution of known volume and concentration
- volume of solution required to dissolve a compound of known mass to a desired concentration
- concentration of a solution resulting from a known mass of compound in a specific volume