Erythrodiol is a natural product found in Salacia chinensis, Celastrus kusanoi, and other organisms with data available.
See also: Calendula Officinalis Flower (part of); Centaurium erythraea whole (part of).
Erythrodiol
CAS No.: 545-48-2
Cat. No.: VC21341370
Molecular Formula: C30H50O2
Molecular Weight: 442.7 g/mol
* For research use only. Not for human or veterinary use.
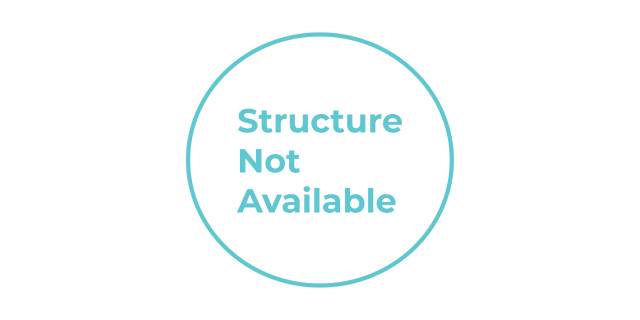
CAS No. | 545-48-2 |
---|---|
Molecular Formula | C30H50O2 |
Molecular Weight | 442.7 g/mol |
IUPAC Name | (3S,4aR,6aR,6bS,8aS,12aS,14aR,14bR)-8a-(hydroxymethyl)-4,4,6a,6b,11,11,14b-heptamethyl-1,2,3,4a,5,6,7,8,9,10,12,12a,14,14a-tetradecahydropicen-3-ol |
Standard InChI | InChI=1S/C30H50O2/c1-25(2)14-16-30(19-31)17-15-28(6)20(21(30)18-25)8-9-23-27(5)12-11-24(32)26(3,4)22(27)10-13-29(23,28)7/h8,21-24,31-32H,9-19H2,1-7H3/t21-,22-,23+,24-,27-,28+,29+,30+/m0/s1 |
Standard InChI Key | PSZDOEIIIJFCFE-OSQDELBUSA-N |
Isomeric SMILES | C[C@]12CC[C@@H](C([C@@H]1CC[C@@]3([C@@H]2CC=C4[C@]3(CC[C@@]5([C@H]4CC(CC5)(C)C)CO)C)C)(C)C)O |
Canonical SMILES | CC1(CCC2(CCC3(C(=CCC4C3(CCC5C4(CCC(C5(C)C)O)C)C)C2C1)C)CO)C |
Chemical Structure and Physical Properties
Erythrodiol features a fused 6-6-6-6-6 pentacyclic triterpenoid moiety with two hydroxyl groups strategically positioned at different locations of the molecular structure . One hydroxyl group (secondary -OH) is attached at the C3 position of the "A" ring, while the other (primary -OH) is located at C28 at the junction of the cis-fused "D" and "E" rings . The molecular weight of erythrodiol is 442.7 g/mol .
The molecule has a β-amyrin type skeleton and can be schematically represented as an unsymmetrical bola-type amphiphile . Computational studies using DFT calculation and Allinger's MMX algorithm have revealed that the molecular length of erythrodiol is approximately 1.57 nm . The lipophilic 6-6-6-6-6 fused pentacyclic backbone combined with polar functional groups at both ends of the nanosized triterpenoid backbone makes erythrodiol a unique amphiphile with distinct self-assembly properties .
Table 1: Physical and Chemical Properties of Erythrodiol
Property | Description |
---|---|
Chemical Name | Erythrodiol; 3β-olean-12-ene-3,28-diol |
Molecular Formula | C30H50O2 |
Molecular Weight | 442.7 g/mol |
Physical Appearance | White crystalline solid |
Molecular Length | 1.57 nm |
Structure Type | Pentacyclic triterpenoid; β-amyrin type skeleton |
Functional Groups | Secondary -OH at C3; Primary -OH at C28 |
Ring System | Fused 6-6-6-6-6 pentacyclic structure |
The solubility of erythrodiol varies in different solvents, as shown in Table 2, highlighting its amphiphilic character.
Table 2: Solubility of Erythrodiol in Various Solvents
Entry | Medium | Concentration (mM) | Solubility State |
---|---|---|---|
1 | o-xylene | 56.5 | Colloidal suspension |
2 | m-xylene | 45.2 | Colloidal suspension |
3 | p-xylene | 45.2 | Colloidal suspension |
4 | mesitylene | 90.4 | Colloidal suspension |
5 | chlorobenzene | 45.2 | Colloidal suspension |
6 | o-dichlorobenzene | 56.5 | Colloidal suspension |
7 | 2-propanol | 45.2 | Colloidal suspension |
8 | EtOH | 22.6 | Soluble |
9 | DMSO | 45.2 | Soluble |
10 | EtOH-H2O (1:1) | 45.2 | Colloidal suspension |
11 | DMSO-H2O (1:1) | 22.6 | Colloidal suspension |
12 | H2O | 22.6 | Insoluble |
Natural Sources and Extraction Methods
Erythrodiol is primarily found in olive oil, particularly in virgin olive oil extracted from Olea europaea . It is also present in the dried leaves of olive trees . Additionally, this compound has been identified in other plant species, including Rhododendron ferrugineum, Salacia chinensis, and Celastrus kusanoi .
There are multiple methods to obtain erythrodiol. One common method is the extraction from dried olive leaves . Alternatively, it can be synthesized through a one-step reduction of oleanolic acid extracted from Lantana camara using LiAlH4, which yields erythrodiol as a white crystalline solid . Another synthesis approach involves the reduction of methyl 3β-acetoxy-12-oxo-oleanoate with LiAlH4, which leads to isomeric erythrodiol 12β- and 12α-hydroxy-derivatives .
The extraction process and methods significantly impact the yield and quality of erythrodiol obtained. Different extraction techniques such as maceration, soxhletation, reflux, and percolation can be employed, with each method affecting the type and quality of the compounds produced . Factors influencing extraction success include the type of solvent, the ratio of solvent to plant material, particle size, temperature, extraction time, and method used .
Recent advancements in extraction technology, such as pulsed electric field (PEF) application, have shown promise in increasing extraction yields. For instance, PEF application resulted in a 17% increase in olive oil yield while maintaining its quality, including the preservation of phytosterols content, which includes compounds like erythrodiol .
Self-Assembly Properties
One of the remarkable properties of erythrodiol is its ability to self-assemble in various organic liquids and aqueous-organic liquid mixtures . This self-assembly behavior results in the formation of diverse architectures at the nano- and microscale.
When introduced into solvents like o-xylene, m-xylene, p-xylene, and mesitylene at concentrations of 2% w/v (45.20 mM), erythrodiol self-assembles to form microsized spherical objects and flower-like structures . In other solvents, it can form nanosized fibrils, microsized flowers, and grass-like architectures through the formation of densely assembled fibrils, petals, or 2D sheets .
The mechanism of self-assembly involves intermolecular hydrogen bonding. FTIR studies have shown that the -OH stretching frequency of neat erythrodiol powder (3354 cm−1) shifts to lower values (3345, 3346, and 3348 cm−1) in dried samples prepared from o-, m-, and p-xylenes . This lowering of -OH stretching frequency indicates stronger intermolecular hydrogen bonding interactions in the self-assemblies, supporting their role during the self-assembly process .
X-ray diffraction studies of erythrodiol self-assemblies prepared from o-xylene, m-xylene, and p-xylene revealed diffraction patterns with peaks at d = 1.57, 0.78, 0.52 nm . These d-values are in the ratio of 1:1/2:1/3, suggesting a lamellar pattern of self-assembly. The optimized molecular length of erythrodiol being 1.57 nm aligns perfectly with this observation .
The proposed model for erythrodiol self-assembly suggests that the molecule, represented as an unsymmetrical bola-type amphiphile, can self-assemble to form linear 1D chains through intermolecular hydrogen bonding . These chains then develop into fibrillar networks, which give rise to petals and long-leaf like architectures, ultimately forming flower- and grass-like morphologies .
Interestingly, these porous self-assemblies, due to their large surface area, demonstrate the ability to adsorb toxic fluorophores like rhodamine-B, rhodamine-6G, methylene blue, and crystal violet, suggesting potential applications in environmental remediation .
Biological Activities
Cardiovascular Effects
Erythrodiol has demonstrated promising effects on cardiovascular health through multiple mechanisms. One significant finding is its ability to increase the half-life of ABCA1 (ATP-binding cassette transporter A1), a key protein involved in cholesterol efflux from macrophages . This effect occurs through inhibition of ABCA1 protein degradation rather than enhancement of its gene transcription .
By stabilizing ABCA1, erythrodiol promotes cholesterol efflux from THP-1-derived human macrophages to lipid-poor apolipoprotein A1 (apoA1) . This process represents the initial step of reverse cholesterol transport, which counteracts the development of atherosclerosis by transporting peripheral cholesterol to the liver for excretion .
Experimental data have shown that erythrodiol at a concentration of 10 μM significantly promotes cholesterol efflux from THP-1 macrophages, comparable to pioglitazone, a well-known macrophage cholesterol efflux enhancer . This effect is specific to ABCA1, as the protein levels of other cholesterol transporters like ABCG1 and SR-B1 remain unaffected by erythrodiol treatment .
Table 3: Effect of Erythrodiol on ABCA1 Protein Degradation
Time After Cycloheximide Addition (min) | ABCA1 Protein Remaining (% of Initial) |
---|---|
Control | |
0 | 100 |
20 | ~85 |
40 | ~70 |
60 | ~50 |
In addition to its effects on cholesterol metabolism, erythrodiol exhibits vasorelaxant activities in aortic rings with functional endothelium . When added accumulatively to aortic rings pre-contracted by 10^-6 M-phenylephrine, erythrodiol induced a maximum relaxation of 73.53% (± 6.01) . This relaxation was significantly attenuated by pre-treatment with the NO synthase inhibitor Nω-nitro-L-arginine-methylester (L-NAME), suggesting that the mechanism is mainly mediated by the endothelial production of nitric oxide (NO) .
Table 4: Vasorelaxant Effects of Erythrodiol on Rat Aorta
Condition | Maximum Relaxation (%) | EC50 (μM) |
---|---|---|
Intact endothelium | 73.53 ± 6.01 | Not specified |
Endothelium-denuded | Almost no effect | - |
With L-NAME (3×10^-4 M) | Significantly attenuated | - |
With indomethacin (10^-5 M) | No significant effect | - |
These cardiovascular effects make erythrodiol a promising compound for protecting the cardiovascular system, and suggest that the intake of "orujo" olive oil, as a source of erythrodiol, might be beneficial in this regard .
Applications
Based on its diverse properties and activities, erythrodiol has several potential applications:
-
Cardiovascular Health: As an ABCA1 stabilizer that promotes cholesterol efflux from macrophages and exhibits vasorelaxant activities, erythrodiol could be developed into a therapeutic agent for cardiovascular diseases, particularly atherosclerosis .
-
Cancer Therapy: The potent antiproliferative and proapoptotic effects of erythrodiol on various cancer cell lines suggest its potential as an anticancer agent or adjuvant therapy .
-
Anti-inflammatory Treatment: Given its reported effectiveness in experimental models of inflammation, erythrodiol might be utilized in developing anti-inflammatory medications .
-
Environmental Remediation: The ability of erythrodiol self-assemblies to adsorb toxic fluorophores like rhodamine-B, rhodamine-6G, methylene blue, and crystal violet suggests potential applications in environmental decontamination .
-
Quality Control: The presence of erythrodiol (along with uvaol) in virgin olive oil is used as a marker for authenticity, helping to identify genuine olive oil products from adulterated ones .
Mass Molarity Calculator
- mass of a compound required to prepare a solution of known volume and concentration
- volume of solution required to dissolve a compound of known mass to a desired concentration
- concentration of a solution resulting from a known mass of compound in a specific volume