Prunasin is a natural product found in Camellia sinensis, Vasconcellea quercifolia, and other organisms with data available.
Prunasin is found in almond. Prunasin is isolated from kernels of Prunus species, immature fruits of Passiflora species and leaves of perilla (Perilla frutescens var. acuta) Prunasin belongs to the family of O-glycosyl Compounds. These are glycosides in which a sugar group is bonded through one carbon to another group via a O-glycosidic bond.
Prunasin
CAS No.: 99-18-3
Cat. No.: VC21341186
Molecular Formula: C14H17NO6
Molecular Weight: 295.29 g/mol
* For research use only. Not for human or veterinary use.
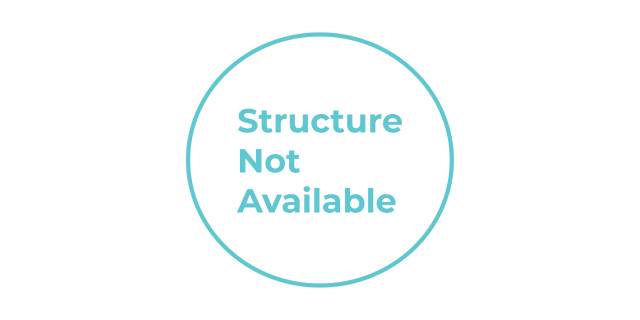
CAS No. | 99-18-3 |
---|---|
Molecular Formula | C14H17NO6 |
Molecular Weight | 295.29 g/mol |
IUPAC Name | (2R)-2-phenyl-2-[(2R,3R,4S,5S,6R)-3,4,5-trihydroxy-6-(hydroxymethyl)oxan-2-yl]oxyacetonitrile |
Standard InChI | InChI=1S/C14H17NO6/c15-6-9(8-4-2-1-3-5-8)20-14-13(19)12(18)11(17)10(7-16)21-14/h1-5,9-14,16-19H,7H2/t9-,10+,11+,12-,13+,14+/m0/s1 |
Standard InChI Key | ZKSZEJFBGODIJW-GMDXDWKASA-N |
Isomeric SMILES | C1=CC=C(C=C1)[C@H](C#N)O[C@H]2[C@@H]([C@H]([C@@H]([C@H](O2)CO)O)O)O |
Canonical SMILES | C1=CC=C(C=C1)C(C#N)OC2C(C(C(C(O2)CO)O)O)O |
Melting Point | 147 - 148 °C |
Chemical Properties and Structure
Molecular Characteristics
Prunasin is defined by specific chemical attributes that determine its stability, reactivity, and biological functions. The compound is identified by the CAS registry number 99-18-3 and possesses the molecular formula C₁₄H₁₇NO₆, corresponding to a molecular weight of 295.29 g/mol . Its structure features a glucose moiety connected via glycosidic linkage to (R)-mandelonitrile, creating the characteristic cyanogenic glycoside framework essential for its biological activity .
Physical Properties
The physical properties of prunasin determine its behavior in biological systems and laboratory settings. These properties are summarized in the following table:
The compound must be stored under specific conditions (−20°C, under inert atmosphere) due to its hygroscopic nature, which can affect its stability and chemical integrity over time . This sensitivity to environmental conditions has implications for both research applications and natural biological contexts.
Natural Occurrences
Distribution in Plant Species
Prunasin demonstrates a distinctive distribution pattern across plant families, with particular concentration in the Rosaceae family. It is abundantly found in various species of the Prunus genus including Prunus japonica, Prunus maximowiczii, and bitter almonds (Prunus dulcis) . The compound is also present in Olinia species, specifically in the leaves and stems of Olinia ventosa, Olinia radiata, Olinia emarginata, and Olinia rochetiana . Additionally, prunasin has been detected in Acacia greggii, demonstrating its distribution beyond the Rosaceae family .
An interesting dietary source of prunasin is dandelion coffee, a coffee substitute derived from dandelion roots, suggesting the compound's presence in the Asteraceae family as well . The widespread but selective distribution of prunasin across plant taxa suggests evolutionary significance in its production and maintenance.
Related Compounds and Stereochemistry
Prunasin exists alongside related compounds that share structural similarities but differ in stereochemical configuration. Most notably, sambunigrin represents a diastereomer of prunasin, derived from (S)-mandelonitrile rather than the (R)-isomer that characterizes prunasin . Sambunigrin has been isolated from the elder tree (Sambucus nigra), where it occurs in a specific ratio with prunasin – 1:3 in leaves and stems, and 2:5 in immature seeds, though it is notably absent in root tissues . This stereochemical variation highlights the specificity of biosynthetic pathways in different plant lineages.
Biosynthesis
Biosynthetic Pathway
The biosynthesis of prunasin follows a well-defined pathway that begins with the amino acid phenylalanine, a product of the shikimate pathway in primary plant metabolism . The conversion process involves several enzymatic steps catalyzed by specialized enzymes:
-
Initial conversion of L-phenylalanine to mandelonitrile, mediated by two cytochrome P450 enzymes: CYP79D16 and CYP71AN24 (characterized in Japanese apricot, Prunus mume)
-
Conversion of mandelonitrile to prunasin, catalyzed by the UDP-glucosyltransferase UGT85A19 (also known as UGT1)
-
Potential further conversion of prunasin to amygdalin by an additional, currently unidentified glucosyltransferase (referred to as UGT2)
This pathway demonstrates how plants channel primary metabolites into specialized defense compounds through coordinated enzymatic activities. The conservation of cytochrome P450 enzymes responsible for prunasin generation across Prunus species suggests evolutionary importance of this biosynthetic capability .
Genetic Regulation
Research has established a correlation between prunasin biosynthesis and genotypic variations in almonds. The accumulation (or lack thereof) of prunasin and amygdalin in almond kernels determines their bitter or sweet phenotype . This genetic control has significant agricultural implications, as almond growers have historically selected for genotypes that minimize amygdalin biosynthesis to produce commercially desirable sweet almonds . Interestingly, high concentrations of prunasin in vegetative regions of almond plants correlate with sweet kernel phenotypes, highlighting the complex relationship between localized and tissue-specific metabolite production .
Biological Functions
Plant Defense Mechanisms
Prunasin serves as a critical component in plant chemical defense systems. It functions within a two-component defense mechanism that is activated upon tissue damage, such as herbivore feeding . This system involves the sequential action of specific hydrolytic enzymes:
-
Prunasin hydrolase (PH) converts prunasin into mandelonitrile and glucose
-
Mandelonitrile lyase 1 (MDL1) catalyzes the dissociation of mandelonitrile into benzaldehyde and hydrogen cyanide
The resulting compounds – bitter benzaldehyde and toxic hydrogen cyanide – create an effective deterrent against herbivores and pathogens . This sophisticated defense strategy represents an evolutionary adaptation that allows plants to store potentially toxic compounds in a stable, non-toxic form until they are needed.
Developmental Regulation
Beyond defense, prunasin plays significant roles in plant developmental processes. Research with almond and sweet cherry has demonstrated that prunasin accumulates in flower buds shortly after dormancy release, with levels subsequently dropping just before flowering . This temporal pattern of accumulation and metabolism suggests prunasin's involvement in controlling flower development in Prunus species .
The turnover of prunasin coincides with increased levels of prunasin amide and other derivatives such as prunasin anitrile pentoside and β-D-glucose-1-benzoate at specific developmental stages . These findings indicate that prunasin may function as a mobile nitrogen-containing compound that contributes to developmental regulation through precisely timed metabolic transformations.
Enzyme Inhibition
Prunasin demonstrates inhibitory effects on specific enzymes that may contribute to its biological functions. Research has shown that (R)-prunasin inhibits rat DNA polymerase β, an enzyme involved in base excision repair required for DNA maintenance and replication, with an IC50 value of 98 μM . This inhibitory capacity may relate to prunasin's effects on plant growth regulation, as observed in several plant systems .
Metabolism and Detoxification
Cyanide Release and Toxicity
The potential toxicity of prunasin stems from its ability to release hydrogen cyanide upon enzymatic hydrolysis. This process, while beneficial for plant defense, presents potential hazards for organisms consuming plant tissues containing high levels of cyanogenic glycosides . The release mechanism requires specific β-glucosidases that hydrolyze the glycosidic bond, followed by hydroxynitrile lyases that catalyze the decomposition of the resulting cyanohydrin .
Detoxification Pathways
Plants containing prunasin have evolved sophisticated detoxification pathways to prevent self-poisoning. The primary detoxification mechanism involves β-cyanoalanine synthase (β-CAS), which catalyzes the conversion of hydrogen cyanide into β-cyanoalanine . Subsequently, type 4 nitrilase catalyzes the hydration of β-cyanoalanine, resulting in the production of asparagine or aspartate and ammonia .
Research has also identified two additional endogenous turnover pathways for cyanogenic glucosides that allow plants to recover nitrogen from these compounds without releasing hydrogen cyanide . These alternative pathways transform prunasin into derivatives such as prunasin amide, which appears during specific developmental stages and may serve additional physiological functions .
Research Applications and Future Directions
Agricultural Implications
Understanding prunasin metabolism has significant implications for agriculture, particularly in crop breeding. The correlation between prunasin content in vegetative tissues and almond kernel sweetness represents valuable knowledge for breeding programs aiming to develop improved almond varieties . Similar applications may extend to other economically important Prunus species like cherries, peaches, and plums.
Biochemical Research Tools
Prunasin serves as an important research tool in understanding plant secondary metabolism and defense mechanisms. Its well-characterized biosynthetic pathway provides insight into how plants produce specialized metabolites from primary precursors . Additionally, the inhibitory effects of prunasin on DNA polymerase β suggest potential applications in biochemical research focused on understanding enzyme inhibition mechanisms and DNA repair processes .
Future Research Directions
Several areas warrant further investigation regarding prunasin:
-
Elucidation of the complete set of enzymes involved in prunasin metabolism, particularly the unidentified glucosyltransferase (UGT2) that converts prunasin to amygdalin
-
Detailed characterization of how prunasin and its derivatives influence specific developmental processes in plants, especially flowering time regulation
-
Investigation of potential bioactive properties of prunasin derivatives that could have pharmacological applications
-
Development of analytical methods to quantify prunasin in diverse food sources to better understand dietary exposure and potential health implications
Mass Molarity Calculator
- mass of a compound required to prepare a solution of known volume and concentration
- volume of solution required to dissolve a compound of known mass to a desired concentration
- concentration of a solution resulting from a known mass of compound in a specific volume