Amorphadiene
CAS No.: 92692-39-2
Cat. No.: VC21340605
Molecular Formula: C15H24
Molecular Weight: 204.35 g/mol
* For research use only. Not for human or veterinary use.
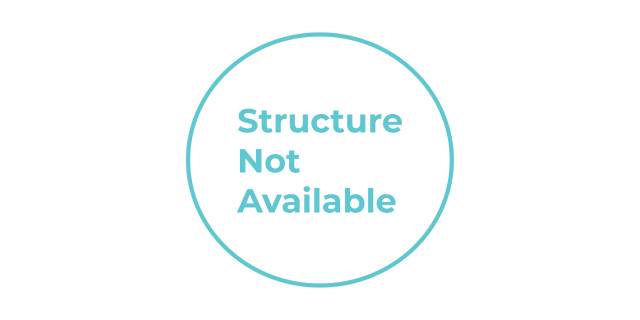
CAS No. | 92692-39-2 |
---|---|
Molecular Formula | C15H24 |
Molecular Weight | 204.35 g/mol |
IUPAC Name | (1R,4R,4aS,8aR)-4,7-dimethyl-1-prop-1-en-2-yl-1,2,3,4,4a,5,6,8a-octahydronaphthalene |
Standard InChI | InChI=1S/C15H24/c1-10(2)13-8-6-12(4)14-7-5-11(3)9-15(13)14/h9,12-15H,1,5-8H2,2-4H3/t12-,13+,14+,15-/m1/s1 |
Standard InChI Key | HMTAHNDPLDKYJT-CBBWQLFWSA-N |
Isomeric SMILES | C[C@@H]1CC[C@H]([C@@H]2[C@H]1CCC(=C2)C)C(=C)C |
SMILES | CC1CCC(C2C1CCC(=C2)C)C(=C)C |
Canonical SMILES | CC1CCC(C2C1CCC(=C2)C)C(=C)C |
Chemical Properties and Structure
Amorphadiene (CAS#: 92692-39-2) is a sesquiterpene with the molecular formula C₁₅H₂₄ and a molecular weight of 204.35100 g/mol. Its IUPAC name is (1R,4R,4aS,8aR)-4,7-dimethyl-1-prop-1-en-2-yl-1,2,3,4,4a,5,6,8a-octahydronaphthalene . The compound contains a bicyclic structure with a characteristic diene system that contributes to its reactivity and biological significance.
Table 1. Physical and Chemical Properties of Amorphadiene
Property | Value |
---|---|
Molecular Formula | C₁₅H₂₄ |
Molecular Weight | 204.35100 g/mol |
Density | 0.9±0.1 g/cm³ |
Boiling Point | 271.9±10.0 °C at 760 mmHg |
Flash Point | 106.6±13.8 °C |
LogP | 6.60 |
Vapor Pressure | 0.0±0.3 mmHg at 25°C |
Index of Refraction | 1.478 |
This bicyclic sesquiterpene serves as the first committed intermediate in the artemisinin biosynthetic pathway, making it a compound of substantial interest for antimalarial drug development and production .
Biosynthesis of Amorphadiene
Natural Production
Amorphadiene is naturally produced in the plant Artemisia annua (sweet wormwood), where it represents the first committed step in artemisinin biosynthesis . The compound's production is tissue-specific, with expression levels of the biosynthetic enzyme being 16-fold higher in leaves than in roots and 10-fold higher than in stems of the plant .
Enzymatic Catalysis
The biosynthesis of amorphadiene is catalyzed by the enzyme amorpha-4,11-diene synthase (ADS), which converts farnesyl pyrophosphate (FPP) to amorphadiene through a complex cyclization reaction . This transformation represents the rate-limiting step in artemisinin biosynthesis and has been the focus of significant research to enhance production efficiency.
The reaction catalyzed by ADS can be represented as:
(2E,6E)-farnesyl diphosphate ⇌ amorpha-4,11-diene + diphosphate
ADS Enzyme Properties
Amorpha-4,11-diene synthase is a 533 amino acid protein with distinctive catalytic properties that influence amorphadiene production.
Table 2. Properties of Amorpha-4,11-diene Synthase (ADS)
Property | Characteristic |
---|---|
Molecular Weight | 62.2 kDa |
Isoelectric Point | 5.25 |
Optimal pH | 6.5 |
Minimum Activity pH | 7.5 |
Required Cofactors | Mg²⁺, Mn²⁺, Co²⁺ (high activity) |
Low Activity Cofactors | Ni²⁺ |
Inactive Cofactors | Cu²⁺, Zn²⁺ |
Protein Length | 533 amino acids |
Conserved Motif | Aspartate-rich DDxxD (substrate binding) |
Recent research has illuminated the catalytic mechanism of ADS, revealing that specific active site residues play crucial roles in the cyclization process. R262 constrains the released pyrophosphate group along with magnesium ions, while aromatic residues (W271, Y519, and F525) stabilize intermediate carbocations. Additionally, T296, G400, G439, and L515 facilitate the 1,6- and 1,10-ring closures essential for amorphadiene formation .
While amorphadiene is the primary product, purified ADS has been shown to produce at least 16 different compounds, highlighting the enzyme's complex reaction mechanisms .
Biotechnological Production of Amorphadiene
Given the critical role of amorphadiene in artemisinin synthesis and the challenges associated with plant-based artemisinin production, significant research has focused on developing microbial systems for amorphadiene biosynthesis.
Production in Escherichia coli
Researchers have engineered E. coli to produce high levels of amorphadiene using a heterologous mevalonate pathway derived from yeast (Saccharomyces cerevisiae). By replacing yeast genes for HMG-CoA synthase and HMG-CoA reductase with equivalent genes from Staphylococcus aureus, production was more than doubled. Further optimization of nitrogen delivery in the fermentation process resulted in consistently high cell densities (90 g/L dry cell weight) and an average titer of 27.4 g/L amorphadiene .
Production in Saccharomyces cerevisiae
Similarly, S. cerevisiae has been engineered for amorphadiene production by overexpressing every enzyme in the mevalonate pathway. Researchers found that CEN.PK2 strains produced amorpha-4,11-diene at concentrations 10-fold higher than artemisinic acid, leading to a focus on amorphadiene production. Through optimization of fermentation conditions, including growth restriction through phosphate limitation and the use of ethanol as a growth substrate, consistent production of over 30 g/L amorphadiene was achieved, with maximal production exceeding 40 g/L .
Production in Bacillus subtilis
Recent studies have demonstrated amorphadiene production in B. subtilis through several innovative approaches:
-
Upregulation of amorphadiene synthase (ADS) expression through fusion with green fluorescent protein (GFP)
-
Co-expression of ADS with a synthetic operon carrying the 2-C-methyl-D-erythritol-4-phosphate (MEP) pathway genes
-
Expression of farnesyl pyrophosphate synthase (FPPS) to ensure sufficient substrate availability
These engineering strategies, combined with experimental design-aided systematic medium optimization, resulted in an amorphadiene yield of 416 ± 15 mg/L. This represents a 20-fold improvement over previous reports in B. subtilis and more than double the production achieved in E. coli or S. cerevisiae at the shake flask fermentation level .
Table 3. Comparison of Amorphadiene Production in Different Organisms
Enzyme Engineering for Enhanced Production
Research into improving amorphadiene production has included significant efforts in enzyme engineering, particularly focused on enhancing the catalytic efficiency of ADS.
Mutability landscape guided enzyme engineering has identified key mutations that substantially improve catalytic activity. For example, the H448A variant showed approximately 4-fold increase in catalytic efficiency, while the double mutation T399S/H448A improved kcat by 5 times . These engineered variants offer promising approaches to enhance amorphadiene production and, consequently, artemisinin biosynthesis.
Additionally, the ADS Q518L variant was found to generate β-copaene in addition to amorphadiene, suggesting that ADS employs both initial 1,6 and 1,10 cyclization mechanisms to produce amorphadiene . This insight into the enzyme's mechanistic flexibility provides further opportunities for optimizing production through targeted engineering.
Applications in Artemisinin Production
Semi-synthetic Artemisinin Production
The high-level production of amorphadiene through microbial fermentation represents a significant milestone in the development of an economically viable process for semi-synthetic artemisinin production. This approach has the potential to substantially increase artemisinin supply for Artemisinin-based Combination Therapies (ACTs), addressing the enormous problem of drug-resistant malaria in developing countries .
Chemical Conversion to Artemisinin Precursors
The conversion of amorphadiene to artemisinin involves multiple oxidation steps. While biological oxidation using the cytochrome P450 enzyme CYP71AV1 has been demonstrated, chemical processes have shown greater efficiency.
A regioselective palladium-catalyzed allylic oxidation of amorphadiene has been developed as a substitute for the P450 enzymes involved in artemisinin biosynthesis. This catalytic step opens new opportunities to supplement a critical step in the current semi-synthetic route and increase the potential of the fermentation process .
Similarly, researchers have developed chemical processes to convert amorphadiene to dihydroartemisinic acid, which can then be converted to artemisinin. These chemical conversions potentially enable industrial-scale production of semi-synthetic artemisinin from microbially produced amorphadiene .
Future Perspectives
The continued optimization of amorphadiene production represents a promising avenue for addressing the global demand for artemisinin. Several research directions show particular promise:
-
Further enzyme engineering of ADS to enhance catalytic efficiency and selectivity
-
Optimization of fermentation processes to increase yields and reduce costs
-
Development of more efficient chemical processes for converting amorphadiene to artemisinin
-
Integration of biological and chemical approaches in hybrid production systems
The significant advances in amorphadiene production achieved through metabolic engineering of microorganisms offer a compelling alternative to plant-derived artemisinin, potentially providing a more stable and scalable supply of this essential antimalarial compound.
Mass Molarity Calculator
- mass of a compound required to prepare a solution of known volume and concentration
- volume of solution required to dissolve a compound of known mass to a desired concentration
- concentration of a solution resulting from a known mass of compound in a specific volume