alpha-Amanitin
CAS No.: 23109-05-9
Cat. No.: VC21340452
Molecular Formula: C39H54N10O14S
Molecular Weight: 919.0 g/mol
* For research use only. Not for human or veterinary use.
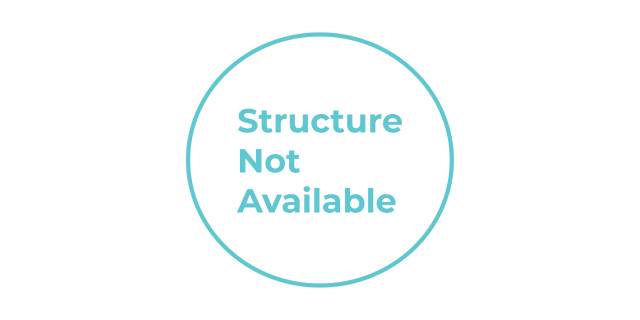
CAS No. | 23109-05-9 |
---|---|
Molecular Formula | C39H54N10O14S |
Molecular Weight | 919.0 g/mol |
IUPAC Name | 2-[(1R,4S,8R,10S,13S,16S,27S,34S)-34-[(2S)-butan-2-yl]-13-[(2R,3R)-3,4-dihydroxybutan-2-yl]-8,22-dihydroxy-2,5,11,14,27,30,33,36,39-nonaoxo-27λ4-thia-3,6,12,15,25,29,32,35,38-nonazapentacyclo[14.12.11.06,10.018,26.019,24]nonatriaconta-18(26),19(24),20,22-tetraen-4-yl]acetamide |
Standard InChI | InChI=1S/C39H54N10O14S/c1-4-16(2)31-36(60)42-11-29(55)43-25-15-64(63)38-21(20-6-5-18(51)7-22(20)46-38)9-23(33(57)41-12-30(56)47-31)44-37(61)32(17(3)27(53)14-50)48-35(59)26-8-19(52)13-49(26)39(62)24(10-28(40)54)45-34(25)58/h5-7,16-17,19,23-27,31-32,46,50-53H,4,8-15H2,1-3H3,(H2,40,54)(H,41,57)(H,42,60)(H,43,55)(H,44,61)(H,45,58)(H,47,56)(H,48,59)/t16-,17-,19+,23-,24-,25-,26-,27-,31-,32-,64-/m0/s1 |
Standard InChI Key | CIORWBWIBBPXCG-YFYLCZILSA-N |
Isomeric SMILES | CC[C@H](C)[C@H]1C(=O)NCC(=O)N[C@H]2C[S@](=O)C3=C(C[C@@H](C(=O)NCC(=O)N1)NC(=O)[C@@H](NC(=O)[C@@H]4C[C@H](CN4C(=O)[C@@H](NC2=O)CC(=O)N)O)[C@@H](C)[C@H](CO)O)C5=C(N3)C=C(C=C5)O |
SMILES | CCC(C)C1C(=O)NCC(=O)NC2CS(=O)C3=C(CC(C(=O)NCC(=O)N1)NC(=O)C(NC(=O)C4CC(CN4C(=O)C(NC2=O)CC(=O)N)O)C(C)C(CO)O)C5=C(N3)C=C(C=C5)O |
Canonical SMILES | CCC(C)C1C(=O)NCC(=O)NC2CS(=O)C3=C(CC(C(=O)NCC(=O)N1)NC(=O)C(NC(=O)C4CC(CN4C(=O)C(NC2=O)CC(=O)N)O)C(C)C(CO)O)C5=C(N3)C=C(C=C5)O |
Colorform | NEEDLES FROM METHANOL |
Melting Point | 254-255 °C |
Chemical Structure and Properties
Alpha-amanitin possesses a unique chemical structure that contributes to its remarkable biological activity. It is a highly modified bicyclic octapeptide with a complex structure consisting of an outer and inner loop .
Structural Components
The outer loop of α-amanitin is formed by conventional peptide bonds between carboxyl and amino termini of adjacent amino acids. The inner loop features a distinctive tryptathionine linkage between 6-hydroxy-tryptophan and cysteine . The toxin's structure is further enhanced by modified amino acid side chains, including (2S,3R,4R)-4,5-dihydroxy-isoleucine and trans-4-hydroxy-proline, which contribute to its high affinity for RNA polymerase II and III .
Physical and Chemical Properties
The table below summarizes the key physical and chemical properties of α-amanitin:
Property | Value |
---|---|
Chemical Formula | C39H54N10O14S |
Molecular Weight | 918.98 g/mol |
Melting Point | 254-255°C |
Boiling Point | 1622.2±65.0°C (Predicted) |
Density | 1.1626 (rough estimate) |
Optical Activity | +191° (alpha D20) |
Water Solubility | Soluble to 5 mM |
pKa | 9.63±0.70 (Predicted) |
Form | White to light yellow powder |
Extinction Coefficient | 13,500 at 310nm in H2O at 1M |
These properties, derived from detailed chemical analyses, highlight α-amanitin's relatively high stability and water solubility, characteristics that contribute to its effectiveness as a toxin .
Sources and Natural Occurrence
Alpha-amanitin occurs naturally in several species of mushrooms, with Amanita phalloides (death cap) being the most notorious source.
Fungal Sources
The toxin is found in multiple mushroom genera:
-
Amanita species, including A. phalloides (death cap) and the destroying angel complex (primarily A. virosa and A. bisporigera)
-
Galerina marginata
-
Lepiota subincarnata
Biosynthesis
Unlike most cyclic peptides, α-amanitin and related amatoxins are ribosomally synthesized rather than produced by non-ribosomal peptide synthetases. The genes encoding the proprotein for α-amanitin belong to the same family as those that encode for phallacidin (a phallotoxin) . This unusual biosynthetic pathway represents a fascinating example of how nature produces highly complex toxic compounds.
Mechanism of Action
The primary molecular mechanism of α-amanitin toxicity involves the selective inhibition of RNA polymerase II (RNAP II), though it also affects RNA polymerase III at higher concentrations.
Interaction with RNA Polymerase II
Alpha-amanitin binds to RNA polymerase II with high affinity, specifically targeting the largest subunit (RPB1) . The crystal structure of the α-amanitin-RNA polymerase II complex, determined at 2.8 Å resolution, has provided crucial insights into this interaction. The toxin binds beneath a "bridge helix" that extends across the cleft between the two largest pol II subunits, Rpb1 and Rpb2, in a "funnel"-shaped cavity .
Key interactions include:
-
A strong hydrogen bond between hydroxyproline 2 of α-amanitin and bridge helix residue Glu-A822
-
An indirect interaction involving the backbone carbonyl group of 4,5-dihydroxyisoleucine 3 of α-amanitin, hydrogen-bonded to residue Gln-A768
-
Several hydrogen bonds between α-amanitin and regions of Rpb1 adjacent to the bridge helix
RNA Polymerase Type | Sensitivity to α-Amanitin |
---|---|
RNA Polymerase I | Insensitive |
RNA Polymerase II | Highly sensitive (inhibited at 1μg/ml) |
RNA Polymerase III | Moderately sensitive (inhibited at 10μg/ml) |
RNA Polymerase IV | Slightly sensitive (inhibited at 50μg/ml) |
This differential sensitivity makes α-amanitin a valuable tool for distinguishing between RNA polymerase types in research settings .
Toxicity and Poisoning
Alpha-amanitin is extremely toxic, with multiple mechanisms contributing to its lethal effects on organisms.
Clinical Manifestations
Amanita poisoning, primarily caused by α-amanitin, has a high mortality rate. The clinical course typically progresses through several phases:
-
Initial gastrointestinal symptoms (6-24 hours after ingestion)
-
Apparent recovery phase
Cellular and Molecular Mechanisms of Toxicity
While inhibition of RNA polymerase II is the primary mechanism of α-amanitin toxicity, several additional pathways contribute to cellular damage:
-
Inhibition of Transcription: By binding to RNA polymerase II, α-amanitin blocks the synthesis of mRNA, leading to a deficit in protein production essential for cell survival .
-
Degradation of RNA Polymerase II: Studies have shown that exposure to α-amanitin triggers degradation of the RPB1 subunit of RNA polymerase II, while other subunits remain relatively unaffected. This degradation appears to be a direct consequence of α-amanitin binding to RPB1 rather than a general activation of proteolytic systems .
-
Apoptosis, Oxidative Stress, and Autophagy: Research has identified apoptosis, oxidative stress, and autophagy as key mechanisms of α-amanitin-induced cell damage .
-
N-Glycan Biosynthesis Pathway: Recent genome-wide CRISPR screening has identified that the N-glycan biosynthesis pathway and its key component, STT3B, play a crucial role in α-amanitin toxicity .
Organ-Specific Effects
Alpha-amanitin exhibits multi-organ toxicity, with the liver and kidneys being particularly vulnerable:
-
Hepatotoxicity: The liver is a major target of α-amanitin, with toxicity manifesting as severe hepatocellular damage and potentially leading to acute liver failure .
-
Nephrotoxicity: Studies in mouse models have demonstrated significant renal injury following α-amanitin exposure, with evidence suggesting a relationship between kidney damage and oxidative stress .
Therapeutic Applications
Despite its extreme toxicity, α-amanitin has attracted interest for therapeutic applications, particularly in the field of targeted cancer therapy.
Antibody-Drug Conjugates
The most promising therapeutic application of α-amanitin is its use as a payload in antibody-drug conjugates (ADCs). These ingeniously designed therapeutics combine:
This approach leverages α-amanitin's potent inhibition of RNA polymerase II to selectively kill cancer cells while sparing normal tissues. Recent preclinical experiments on ADCs incorporating α-amanitin have shown promising therapeutic effects and good tolerability in primate models .
Detection Methods
The detection of α-amanitin is crucial for both clinical diagnosis of mushroom poisoning and research applications.
Analytical Techniques
Several methods have been developed for the detection and quantification of α-amanitin:
-
Thin-layer chromatography (TLC): Early methods could differentiate between alpha-amanitin and beta-amanitin based on their different migration rates in various solvent systems
-
More advanced techniques include high-performance liquid chromatography (HPLC) and mass spectrometry
-
Immunoassays utilizing specific antibodies against α-amanitin
These methods vary in sensitivity, specificity, and applicability to different sample types, including mushroom extracts, biological fluids, and tissues .
Mass Molarity Calculator
- mass of a compound required to prepare a solution of known volume and concentration
- volume of solution required to dissolve a compound of known mass to a desired concentration
- concentration of a solution resulting from a known mass of compound in a specific volume