Lumazine is a natural product found in Formica polyctena with data available.
Lumazine
CAS No.: 487-21-8
Cat. No.: VC21339120
Molecular Formula: C6H4N4O2
Molecular Weight: 164.12 g/mol
* For research use only. Not for human or veterinary use.
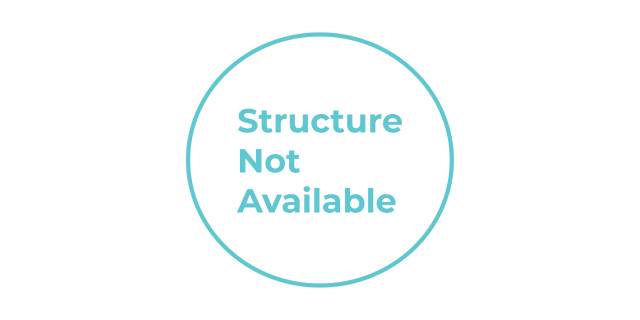
CAS No. | 487-21-8 |
---|---|
Molecular Formula | C6H4N4O2 |
Molecular Weight | 164.12 g/mol |
IUPAC Name | 1H-pteridine-2,4-dione |
Standard InChI | InChI=1S/C6H4N4O2/c11-5-3-4(8-2-1-7-3)9-6(12)10-5/h1-2H,(H2,8,9,10,11,12) |
Standard InChI Key | UYEUUXMDVNYCAM-UHFFFAOYSA-N |
SMILES | C1=CN=C2C(=N1)C(=O)NC(=O)N2 |
Canonical SMILES | C1=CN=C2C(=N1)C(=O)NC(=O)N2 |
Melting Point | 348.5 °C |
Chemical Structure and Classification
Lumazine (2,3,4,8-tetrahydropteridine-2,4-dione) belongs to the class of organic compounds known as pteridines and derivatives. These polycyclic aromatic compounds contain a pteridine moiety, consisting of a pyrimidine ring fused to a pyrazine ring to form pyrimido(4,5-b)pyrazine . The chemical formula of lumazine is C6H4N4O2, with an average molecular weight of 164.1216 and a monoisotopic molecular weight of 164.033425392 . Lumazine is also known by its alternative name 2,4(3H,8H)-pteridinedione and has been assigned the CAS Number 487-21-8 .
Molecular Structure
The molecular structure of lumazine features a bicyclic system with two carbonyl groups at positions 2 and 4. This arrangement contributes to its unique chemical properties and interactions within biological systems. The compound's structure can be represented by the IUPAC name 2,3,4,8-tetrahydropteridine-2,4-dione and the SMILES notation O=C1NC(=O)C2=NC=CNC2=N1 .
Physicochemical Properties
Lumazine exhibits several notable physicochemical properties that influence its behavior in biological systems. It is slightly soluble in water and functions as a very weakly acidic compound based on its pKa values . The compound's water solubility is approximately 3.66 g/L, with a logP value of -0.828, indicating hydrophilic characteristics . The following table summarizes the key physicochemical properties of lumazine:
These properties significantly influence lumazine's interactions with biological macromolecules and its distribution within biological systems.
Biological Distribution and Significance
Lumazine has been detected in various biological sources, offering insights into its potential roles in metabolic pathways and as a biomarker for specific dietary exposures.
Presence in Food Sources
Lumazine has been detected in soy beans (Glycine max), making it a potential biomarker for the consumption of this food product . This association with specific food sources suggests that measuring lumazine levels could potentially be used to assess dietary patterns involving soy consumption, offering applications in nutritional research and dietary assessment.
Lumazine Protein Structure and Function
Lumazine protein (LumP) represents a specialized biological application of lumazine derivatives, playing crucial roles in bacterial bioluminescence systems.
Structural Features
Lumazine protein is a fluorescent accessory protein with 6,7-dimethyl-8-(1′-d-ribityl) lumazine (DMRL) as its authentic chromophore . Crystallographic studies have revealed that LumP consists of two β barrels with nearly identical folds, described as N-terminal and C-terminal barrels . This structural arrangement creates specific binding pockets for lumazine derivatives and related compounds.
The crystal structures of LumP in complex with various ligands, including DMRL, riboflavin (RBF), and flavin mononucleotide (FMN), have been determined at resolutions of 2.00, 1.42, and 2.00 Å respectively . These structural analyses provide detailed insights into the binding mechanisms and functional relationships between lumazine proteins and their chromophores.
Crystallographic Data
Detailed crystallographic studies have provided precise measurements of LumP structures with different ligands, as summarized in the following table:
Characteristic | LumP-RBF | LumP-FMN | LumP-DMRL |
---|---|---|---|
Temperature (K) | 100 | 95 | 95 |
Resolution (Å) | 1.42 | 2.00 | 2.00 |
Space group | P2₁2₁2₁ | P2₁2₁2₁ | P2₁2₁2₁ |
Cell dimensions (Å) | |||
a | 46.288 | 46.858 | 46.689 |
b | 46.582 | 47.087 | 46.584 |
c | 161.053 | 160.923 | 161.587 |
R work | 0.192 | 0.196 | 0.195 |
R free | 0.234 | 0.255 | 0.255 |
These crystallographic measurements demonstrate the high precision with which the structures have been determined, enabling detailed analysis of chromophore-protein interactions .
Ligand Binding Mechanism
In all studied structures, the chromophore (whether DMRL, RBF, or FMN) is tethered to a narrow cavity via multiple hydrogen bonds in the N-terminal domain, while such interactions are absent in the C-terminal domain . This asymmetric binding pattern is functionally significant, as it creates a specific environment for the chromophore that influences its spectral properties.
The binding mechanism varies slightly depending on the ligand. For instance, hydrogen bonding in LumP-FMN is decreased compared to LumP-RBF because the phosphate moiety of FMN protrudes out of the narrow cavity . In LumP-DMRL, the side chain of Gln65 positions close to the ring system, and a unique water molecule that stabilizes the ligand is observed near Ser48 . These subtle differences in binding mechanisms contribute to the specific functional properties of each complex.
Role in Bacterial Bioluminescence
Lumazine protein plays a crucial role in modulating bacterial bioluminescence, particularly in marine bacteria of the genus Photobacterium.
Spectral Modulation
Beyond simply altering the emission wavelength, lumazine protein also enhances the intensity of the light produced in bacterial bioluminescence reactions . This dual function of wavelength modulation and intensity enhancement makes lumazine protein an important component in the bioluminescence systems of specific bacterial species.
Energy Transfer Mechanism
The mechanism by which lumazine protein modulates bioluminescence involves the formation of a protein-protein complex between lumazine protein and luciferase . Within this complex, rapid energy transfer occurs between the flavin molecule on the luciferase and the lumazine derivative bound to lumazine protein.
Structural Relationships and Evolutionary Significance
Lumazine protein shares notable structural and functional relationships with other proteins, providing insights into its evolutionary origins and biochemical significance.
Relationship to Riboflavin Synthase
Lumazine protein exhibits a high amino acid sequence homology with riboflavin synthase, an enzyme that generates one riboflavin molecule from two DMRL molecules . This structural similarity suggests evolutionary relationships between these proteins, despite their different functional roles.
Riboflavin synthase exists as a homo-trimer, with each monomer containing two segments with similar sequences and closely related folds, identified as N-terminal and C-terminal domains . This structural arrangement parallels that observed in lumazine protein, though with distinct functional implications. In riboflavin synthase, the catalytic site is proposed to be located at the interface between the N-terminal domain of one subunit and the C-terminal domain of an adjacent subunit .
Evolutionary Implications
The structural similarities between lumazine protein and riboflavin synthase suggest potential evolutionary relationships, where proteins with similar structural scaffolds have evolved to perform distinct functions. Lumazine protein may represent an evolutionary adaptation of the riboflavin synthase fold to serve specific functions in bacterial bioluminescence systems, illustrating the principle of repurposing existing protein structures for novel functions throughout evolutionary history.
Research Methodologies and Techniques
Research on lumazine and lumazine proteins has employed diverse methodologies, providing comprehensive insights into their properties and functions.
Protein Expression and Purification
Research on lumazine protein has utilized sophisticated protein expression and purification techniques. For instance, lumazine protein has been solubilized in urea buffer containing riboflavin, refolded by removing the urea, and purified by column chromatography . The successful binding of ligands to the purified protein has been confirmed through absorbance and fluorescence spectroscopy, demonstrating peaks at 460 nm (absorbance) and 535 nm (fluorescence emission with 445 nm excitation) for riboflavin-bound lumazine protein .
Spectroscopic Analysis
Spectroscopic techniques have been instrumental in characterizing lumazine and lumazine proteins. Absorbance and fluorescence spectroscopy have been used to confirm ligand binding and characterize the spectral properties of lumazine protein complexes . Fluorescence dynamics measurements, particularly the decay of fluorescence anisotropy, have provided insights into the energy transfer mechanisms between lumazine protein and luciferase .
Crystallographic Studies
X-ray crystallography has been employed to determine the three-dimensional structures of lumazine protein in complex with various ligands . These studies have provided detailed information about protein folding, ligand binding mechanisms, and structural relationships between lumazine protein and related proteins such as riboflavin synthase.
Mass Molarity Calculator
- mass of a compound required to prepare a solution of known volume and concentration
- volume of solution required to dissolve a compound of known mass to a desired concentration
- concentration of a solution resulting from a known mass of compound in a specific volume