Tripotassium arsenate
CAS No.: 13464-36-3
Cat. No.: VC20982972
Molecular Formula: AsK3O4
Molecular Weight: 256.214 g/mol
* For research use only. Not for human or veterinary use.
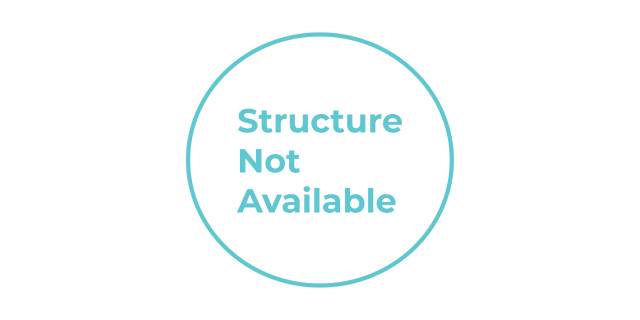
CAS No. | 13464-36-3 |
---|---|
Molecular Formula | AsK3O4 |
Molecular Weight | 256.214 g/mol |
IUPAC Name | tripotassium;arsorate |
Standard InChI | InChI=1S/AsH3O4.3K/c2-1(3,4)5;;;/h(H3,2,3,4,5);;;/q;3*+1/p-3 |
Standard InChI Key | QLFYYVADGRLXQS-UHFFFAOYSA-K |
SMILES | [O-][As](=O)([O-])[O-].[K+].[K+].[K+] |
Canonical SMILES | [O-][As](=O)([O-])[O-].[K+].[K+].[K+] |
Chemical Identity and Properties
Tripotassium arsenate, also known as arsenic acid tripotassium salt, is characterized by its specific chemical composition and unique physical properties that distinguish it from other arsenic-containing compounds.
Basic Identification
Tripotassium arsenate appears as colorless crystals under standard conditions. It carries the CAS registry number 13464-36-3 and is identified within chemical databases through various systematic nomenclatures . The compound is recognized by several synonyms including tripotassium arsenate, arsenic acid tripotassium salt, and potassium arsenate normal, which may appear in different scientific literature and chemical inventories .
Physical and Chemical Properties
The physical and chemical properties of tripotassium arsenate determine its behavior in various environments and applications. These properties are summarized in the following table:
Distinction from Arsenite Compounds
It is crucial to distinguish tripotassium arsenate (K₃AsO₄) from tripotassium arsenite (K₃AsO₃), as these compounds possess different chemical properties and toxicological profiles. Tripotassium arsenate contains arsenic in the pentavalent state (As⁵⁺), while tripotassium arsenite contains arsenic in the trivalent state (As³⁺) . This oxidation state difference significantly affects their chemical behavior and biological interactions.
Synthesis Methods
The preparation of tripotassium arsenate can be accomplished through various synthetic routes, with the most common method involving the reaction between potassium hydroxide and arsenic acid.
Standard Synthesis Procedure
Tripotassium arsenate can be synthesized by reacting potassium hydroxide (KOH) with arsenic acid (H₃AsO₄) in a stoichiometric ratio. The general reaction proceeds as follows:
H₃AsO₄ + 3KOH → K₃AsO₄ + 3H₂O
This neutralization reaction involves the complete deprotonation of arsenic acid by the strong base potassium hydroxide, yielding the tripotassium salt and water as products. The synthesis requires controlled conditions to ensure complete reaction and product purity.
Alternative Preparation Methods
Alternative methods for the preparation of tripotassium arsenate may involve reactions starting from other arsenic-containing compounds. For instance, oxidation of arsenite compounds or reactions involving arsenic pentoxide with potassium-containing reagents can potentially yield tripotassium arsenate under appropriate conditions .
Related Potassium Arsenate Compounds
Tripotassium arsenate belongs to a family of potassium arsenate compounds that differ in their potassium content and degree of protonation of the arsenate anion.
Comparison with Other Potassium Arsenates
The arsenate family includes monopotassium arsenate (KH₂AsO₄) and dipotassium arsenate (K₂HAsO₄), which represent partially deprotonated forms of arsenic acid . The following table compares these compounds:
Acid-Base Behavior
The acid-base behavior of these compounds is related to the dissociation constants of arsenic acid. Relevant acid-base equilibria for aqueous solutions of arsenic acid derivatives include :
-
H₃AsO₄ + H₂O ⇌ H₂AsO₄⁻ + H₃O⁺ (pKa₁ = 2.19)
-
H₂AsO₄⁻ + H₂O ⇌ HAsO₄²⁻ + H₃O⁺ (pKa₂ = 6.94)
-
HAsO₄²⁻ + H₂O ⇌ AsO₄³⁻ + H₃O⁺ (pKa₃ > 11)
Tripotassium arsenate corresponds to the fully deprotonated arsenate anion (AsO₄³⁻) combined with three potassium cations .
Chemical Behavior and Stability
Understanding the chemical behavior and stability of tripotassium arsenate is essential for its proper handling and application.
Solubility and Solution Behavior
Tripotassium arsenate is water-soluble, forming arsenate ions in solution. In aqueous environments, the arsenate ion may undergo various reactions depending on the pH, redox conditions, and presence of other chemical species .
Redox Transformations
Under reducing conditions, pentavalent arsenic species like arsenate can be converted to trivalent forms. This transformation is particularly relevant in environmental and biological contexts, where such redox changes affect arsenic mobility and toxicity :
AsO₄³⁻ + 2e⁻ + 2H⁺ → AsO₃³⁻ + H₂O
Under extremely reducing conditions, arsenic compounds may be further reduced to insoluble sulfides, affecting their environmental fate .
Environmental Behavior
In environmental settings, arsenate compounds like tripotassium arsenate can interact with various components of soil and water systems. Arsenate partitions from water to sediment phases through processes such as precipitation and adsorption . Precipitation may occur with aluminum and iron at low pH and with calcium and magnesium at high pH, while adsorption processes can occur across a broader range of conditions .
Toxicological Considerations
Arsenic compounds, including tripotassium arsenate, are known for their toxicity, which necessitates careful handling and regulatory oversight.
Hazard Classification
Tripotassium arsenate is classified as a hazardous material with UN identification number 1677, falling under hazard class 6.1 (Poisonous Material) and packing group II, indicating a moderate to high level of toxicity . The emergency response guide number associated with this compound is 151 .
Health Effects
Inorganic arsenic compounds can cause various adverse health effects through different exposure routes. Chronic arsenic exposure may lead to multiple systemic effects, including :
-
Dermatological effects: Hyperkeratosis and changes in skin pigmentation
-
Cardiovascular diseases
-
Pulmonary diseases
-
Peripheral neuropathy
-
Developmental and cognitive impairments
-
Carcinogenic changes in multiple organs including skin, liver, lung, bladder, and prostate
The pentavalent form of arsenic found in arsenate compounds may substitute for phosphate ions in biological systems, potentially disrupting biochemical processes.
Toxicity Comparison
Pentavalent arsenic compounds like tripotassium arsenate generally have different toxicity mechanisms compared to trivalent forms. The following table outlines some key differences:
Analytical Detection and Measurement
Various analytical techniques have been developed for the detection and quantification of arsenate compounds in different matrices.
High-Performance Liquid Chromatography (HPLC)
HPLC coupled with inductively coupled plasma mass spectrometry (HPLC-ICP-MS) has been successfully applied for the separation and detection of different arsenic species, including arsenate compounds. This technique allows for the differentiation between arsenite (As³⁺), arsenate (As⁵⁺), monomethylarsonic acid (MMA⁵⁺), and dimethylarsinic acid (DMA⁵⁺) .
A study by Molecules (2019) reported a separation technique for arsenic species with a short run time of 3.7 minutes using an alkyl reverse phase column and highly aqueous mobile phase containing 20 mM citric acid and 5 mM sodium hexanesulfonate (pH = 4.3) . This method achieved calibration curves that were linear over the range of 5–500 ng·mL⁻¹ (measured as As), with correlation coefficients greater than 0.99 .
Sample Preparation Considerations
Sample preparation is critical for accurate analysis of arsenate compounds. The potential interconversion between different arsenic species during sample processing must be considered. For example, arsenite (As³⁺) can be oxidized to arsenate (As⁵⁺) under aerobic conditions. In one study, the addition of ammonium sulfate (0.05 g per 100 µL of plasma) was found to inhibit the conversion of As³⁺ to As⁵⁺ during sample processing .
Applications and Research Directions
The applications of tripotassium arsenate are influenced by its chemical properties and constrained by its toxicity concerns.
Chemical Research
In chemical research, tripotassium arsenate has been used in studies exploring the properties and behavior of arsenate compounds. For instance, research on the K₂O-Bi₂O₃-As₂O₅ system resulted in the isolation of a new arsenate of trivalent bismuth, K₃Bi₅(AsO₄)₆, through solid-state reaction at 600°C .
Ion Conductivity Studies
Some arsenate compounds exhibit ion conductivity properties that are of interest in materials science research. For example, the compound K₃Bi₅(AsO₄)₆ was found to exhibit potassium ion conductivity, although with relatively low conductivity values . Such studies contribute to the broader understanding of ion transport mechanisms in crystalline materials.
Arsenic Metabolism Research
Research into arsenic metabolism has utilized various arsenic compounds to understand the pharmacokinetic properties and transformation of arsenic species in biological systems. Studies have investigated the conversion between different arsenic oxidation states and the formation of methylated metabolites like monomethylarsonic acid (MMA⁵⁺) and dimethylarsinic acid (DMA⁵⁺) .
Mass Molarity Calculator
- mass of a compound required to prepare a solution of known volume and concentration
- volume of solution required to dissolve a compound of known mass to a desired concentration
- concentration of a solution resulting from a known mass of compound in a specific volume