2,2,2-Trideuterioacetic acid
CAS No.: 1112-02-3
Cat. No.: VC20954753
Molecular Formula: C2H4O2
Molecular Weight: 63.07 g/mol
* For research use only. Not for human or veterinary use.
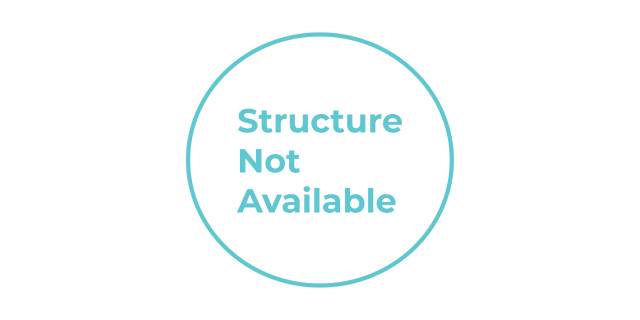
CAS No. | 1112-02-3 |
---|---|
Molecular Formula | C2H4O2 |
Molecular Weight | 63.07 g/mol |
IUPAC Name | 2,2,2-trideuterioacetic acid |
Standard InChI | InChI=1S/C2H4O2/c1-2(3)4/h1H3,(H,3,4)/i1D3 |
Standard InChI Key | QTBSBXVTEAMEQO-FIBGUPNXSA-N |
Isomeric SMILES | [2H]C([2H])([2H])C(=O)O |
SMILES | CC(=O)O |
Canonical SMILES | CC(=O)O |
Chemical Properties and Structure
2,2,2-Trideuterioacetic acid maintains the fundamental structure of acetic acid but with three deuterium atoms replacing the hydrogen atoms in the methyl group. Its molecular formula is C2D3HO2, and it has a molecular weight of 63.07 g/mol, slightly higher than that of regular acetic acid (60.05 g/mol) due to the additional neutrons in the deuterium atoms .
The physical properties of 2,2,2-trideuterioacetic acid closely resemble those of conventional acetic acid. It exists as a liquid at room temperature with similar boiling and melting points to acetic acid, though with slight variations due to isotope effects. The compound remains acidic, with a pKa value comparable to that of regular acetic acid, as the acidic proton in the carboxyl group remains unchanged.
Spectroscopically, 2,2,2-trideuterioacetic acid exhibits distinct characteristics. In infrared spectroscopy, the C-D stretching vibrations occur at lower frequencies (around 2100-2200 cm⁻¹) compared to C-H stretching vibrations (around 2800-3000 cm⁻¹) in regular acetic acid. In NMR spectroscopy, deuterium atoms show different coupling patterns and chemical shifts compared to hydrogen, providing valuable analytical information.
Table 1: Comparison of Key Properties Between Acetic Acid and Its Deuterated Analogs
Synthesis Methods
Several synthetic approaches have been developed for the preparation of 2,2,2-trideuterioacetic acid, each with specific advantages depending on the required purity, scale, and available resources.
Base-Catalyzed Exchange
One common method involves the base-catalyzed exchange of hydrogen atoms in the methyl group of acetic acid with deuterium from deuterium oxide (D₂O). This approach typically employs a catalytic amount of a strong base to facilitate the exchange at the α-position of the carboxylic acid. The reaction proceeds through the formation of an enolate intermediate, which undergoes deuteration upon protonation with D₂O.
Oxidation of Deuterated Precursors
Another approach involves the oxidation of deuterated precursors such as trideuterioacetaldehyde (CD₃CHO) or trideuterioethanol (CD₃CH₂OH). These precursors can be obtained through various deuteration methods, and their subsequent oxidation leads to the formation of 2,2,2-trideuterioacetic acid. This method is particularly valuable when high isotopic purity is required.
Applications in Scientific Research
2,2,2-Trideuterioacetic acid finds numerous applications across various scientific disciplines due to its unique isotopic composition and chemical properties.
Nuclear Magnetic Resonance Spectroscopy
One of the primary applications of 2,2,2-trideuterioacetic acid is in NMR spectroscopy, where it serves as both a deuterated solvent and a reference compound. The deuteration of the methyl group reduces interfering signals while maintaining the acidic environment necessary for certain analyses. It is particularly useful for studying compounds that might interact with or be obscured by regular acetic acid signals .
When used as an NMR solvent, 2,2,2-trideuterioacetic acid is often formulated with small amounts (typically 0.03% v/v) of tetramethylsilane (TMS) as a chemical shift reference standard . This combination provides a versatile medium for analyzing a wide range of organic compounds, particularly those requiring acidic conditions.
Reaction Mechanism Studies
The selective deuteration in 2,2,2-trideuterioacetic acid makes it an excellent tool for investigating reaction mechanisms. By tracking the fate of deuterium atoms during chemical transformations, researchers can gain insights into bond-breaking and bond-forming processes, helping to elucidate reaction pathways and intermediates.
For example, in peptide synthesis and pharmaceutical research, 2,2,2-trideuterioacetic acid has been used to study acid-catalyzed reactions and to identify the specific sites of protonation or deprotonation in complex molecules . These studies provide valuable information about reaction selectivity and efficiency.
Kinetic Isotope Effect Studies
The substitution of hydrogen with deuterium affects the rate of reactions involving C-D bonds compared to C-H bonds, a phenomenon known as the kinetic isotope effect (KIE). 2,2,2-Trideuterioacetic acid serves as a model compound for studying these effects, providing valuable information about reaction transition states and energy barriers.
Comparative studies of reaction rates between regular acetic acid and 2,2,2-trideuterioacetic acid can reveal the importance of methyl hydrogen atoms in the rate-determining step of various reactions. This information is crucial for understanding reaction mechanisms and developing more efficient synthetic methods.
Analytical Chemistry Applications
In analytical chemistry, 2,2,2-trideuterioacetic acid is used as an internal standard or reference compound in mass spectrometry, gas chromatography, and other analytical techniques. The distinctive mass signature resulting from deuteration helps in quantification and identification processes, particularly in complex mixtures where overlapping signals might otherwise complicate analysis.
Environmental and Atmospheric Chemistry
2,2,2-Trideuterioacetic acid has been employed in atmospheric chemistry studies to investigate the fate and transformation of acetic acid in the atmosphere. By comparing the reactivity of deuterated and non-deuterated analogs with atmospheric oxidants such as hydroxyl radicals, researchers gain insights into degradation pathways and atmospheric lifetimes of carboxylic acids .
Kinetic Studies and Reaction Mechanisms
Significant research has been conducted on the kinetics of reactions involving 2,2,2-trideuterioacetic acid, particularly its reactions with hydroxyl radicals. These studies provide valuable insights into reaction mechanisms and the effects of isotopic substitution on reaction rates.
Hydroxyl Radical Reactions
Studies of the reactions between hydroxyl radicals and various deuterated forms of acetic acid have provided crucial information about the mechanisms of these environmentally relevant processes. At 300 K, the rate constant for the reaction of OH with 2,2,2-trideuterioacetic acid (CD₃COOH) was measured to be (7.79 ± 0.16) × 10⁻¹³ cm³ molecule⁻¹ s⁻¹, which is comparable to the rate constant for regular acetic acid (7.42 ± 0.12) × 10⁻¹³ cm³ molecule⁻¹ s⁻¹ .
In contrast, when the acidic hydrogen is also deuterated (as in acetic acid-d₄, CD₃COOD), the rate constant decreases significantly to (1.09 ± 0.18) × 10⁻¹³ cm³ molecule⁻¹ s⁻¹. This substantial difference suggests that the primary mechanism for this reaction involves abstraction of the acidic hydrogen rather than abstraction of the hydrogen atoms in the methyl group .
Table 2: Rate Constants for Reactions of OH with Acetic Acid and Its Deuterated Analogs at 300 K
Compound | Chemical Formula | Rate Constant (cm³ molecule⁻¹ s⁻¹) |
---|---|---|
Acetic Acid | CH₃COOH | (7.42 ± 0.12) × 10⁻¹³ |
2,2,2-Trideuterioacetic Acid | CD₃COOH | (7.79 ± 0.16) × 10⁻¹³ |
Acetic Acid-d₄ | CD₃COOD | (1.09 ± 0.18) × 10⁻¹³ |
Temperature Dependence and Arrhenius Parameters
The temperature dependence of hydroxyl radical reactions with acetic acid and its deuterated analogs provides further insights into reaction mechanisms. The rate constants for both OH + acetic acid and OH + acetic acid-d₄ reactions display a negative temperature dependence, which is relatively unusual for atmospheric oxidation reactions .
This temperature dependence can be described by the following Arrhenius equations:
-
For acetic acid: kₕ(T) = (2.52 ± 1.22) × 10⁻¹⁴ exp((1010 ± 150)/T) cm³ molecule⁻¹ s⁻¹
-
For acetic acid-d₄: kₚ(T) = (4.62 ± 1.33) × 10⁻¹⁶ exp((1640 ± 160)/T) cm³ molecule⁻¹ s⁻¹
The negative temperature dependence suggests that the lifetime of acetic acid at the low temperatures of the upper troposphere may be shorter than previously believed, which has implications for atmospheric chemistry models and our understanding of carboxylic acid cycling in the environment .
Comparative Studies with Other Deuterated Acids
Comparing 2,2,2-trideuterioacetic acid with other deuterated organic acids provides valuable insights into the effects of deuteration patterns on various properties and reactivities.
Comparison with Trifluoroacetic Acid
Studies have compared the properties and reactivities of 2,2,2-trideuterioacetic acid with those of 2,2,2-trifluoroacetic acid (TFA), another substituted acetic acid derivative where the three hydrogen atoms in the methyl group are replaced with fluorine atoms .
While both compounds feature substitution at the methyl position, their properties differ significantly due to the contrasting electronic effects of deuterium and fluorine. Deuterium is electronically similar to hydrogen but has different mass and vibrational characteristics, whereas fluorine is highly electronegative and substantially alters the electronic distribution in the molecule.
These differences manifest in various properties, including acidity (TFA is much more acidic than 2,2,2-trideuterioacetic acid), reactivity patterns, and applications. TFA is often used as a strong acid catalyst and reagent in organic synthesis, while 2,2,2-trideuterioacetic acid is primarily employed as an isotopically labeled compound for mechanistic and analytical studies .
Reactivity Patterns Across Deuterated Variants
Studies comparing the reactivity patterns across different deuterated variants of acetic acid, including 2,2,2-trideuterioacetic acid, acetic acid-d₄, and selectively deuterated variants, provide insights into the site-specific effects of deuteration on chemical behavior.
For example, the comparison of hydroxyl radical reaction rates with different deuterated acetic acids reveals that deuteration at the methyl position (as in 2,2,2-trideuterioacetic acid) has minimal impact on the reaction rate, while deuteration of the acidic hydrogen (as in acetic acid-d₄) significantly reduces the rate . These observations help elucidate the preferential sites of reaction and the mechanisms involved.
Applications in Advanced Synthetic Chemistry
2,2,2-Trideuterioacetic acid plays important roles in advanced synthetic chemistry, particularly in the development of deuterated pharmaceuticals and the incorporation of deuterium labels into complex molecules.
Deuterated Pharmaceuticals Development
The development of deuterated pharmaceuticals, or "heavy drugs," has gained significant attention in recent years due to the potential benefits of deuteration on drug metabolism, pharmacokinetics, and safety profiles. 2,2,2-Trideuterioacetic acid serves as a valuable building block and reagent in the synthesis of such deuterated compounds .
The "deuterated magic methyl" approach, which involves the strategic incorporation of CD₃ groups into drug molecules, has shown promise in modifying drug properties while maintaining efficacy. 2,2,2-Trideuterioacetic acid and its derivatives provide convenient sources of CD₃ units for such applications .
Trideuteromethylation Methodologies
Various methodologies have been developed for site-selective trideuteromethylation using CD₃ reagents derived from or related to 2,2,2-trideuterioacetic acid. These methods enable the precise installation of trideuteromethyl groups in target molecules, which is valuable for drug optimization and development .
Table 3: Selected Trideuteromethylation Reagents and Their Applications
Mass Molarity Calculator
- mass of a compound required to prepare a solution of known volume and concentration
- volume of solution required to dissolve a compound of known mass to a desired concentration
- concentration of a solution resulting from a known mass of compound in a specific volume