Lead(II) sulfide
CAS No.: 1314-87-0
Cat. No.: VC20953938
Molecular Formula: PbS
Molecular Weight: 239 g/mol
* For research use only. Not for human or veterinary use.
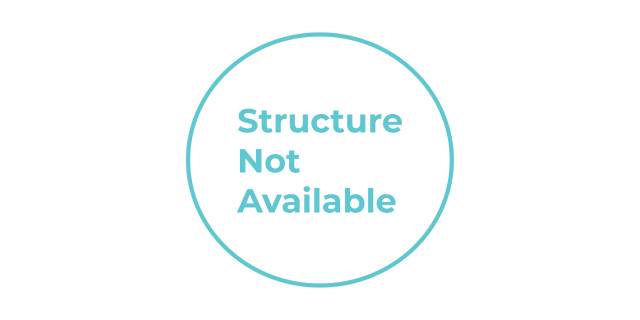
CAS No. | 1314-87-0 |
---|---|
Molecular Formula | PbS |
Molecular Weight | 239 g/mol |
IUPAC Name | sulfanylidenelead |
Standard InChI | InChI=1S/Pb.S |
Standard InChI Key | XCAUINMIESBTBL-UHFFFAOYSA-N |
SMILES | S=[Pb] |
Canonical SMILES | S=[Pb] |
Boiling Point | 2337.8 °F at 760 mm Hg (USCG, 1999) 1281 °C (sublimes) |
Colorform | Silvery, metallic crystals or black powder Metallic black cubic crystals |
Melting Point | 2037.2 °F (USCG, 1999) 1113 °C |
Physical and Chemical Properties
Basic Properties
Lead(II) sulfide exhibits distinctive physical and chemical characteristics that contribute to its technological utility. The following table summarizes its key properties:
Crystal Structure
Lead(II) sulfide crystallizes in a face-centered cubic (FCC) structure, specifically the halite structure with a space group Fm3m . This crystal arrangement is similar to that of sodium chloride, with lead and sulfur atoms occupying alternating positions in the cubic lattice . Unlike many other IV-VI semiconductors, Lead(II) sulfide maintains this sodium chloride motif, which contributes to its distinctive electronic properties .
The cubic crystal structure gives Lead(II) sulfide its characteristic cubic cleavage pattern, which is often visible in natural galena samples . These structural characteristics directly influence its electronic band structure and subsequent optical and electronic behavior .
Solubility and Reactivity
Occurrence and Production
Natural Occurrence as Galena
Lead(II) sulfide occurs naturally as the mineral galena, which is the primary ore of lead worldwide . Galena typically appears as lead-gray cubic crystals with metallic luster and perfect cubic cleavage . The mineral often contains various impurities, including silver, which sometimes makes it an important source of this precious metal as well .
Major deposits of galena are found in various locations globally, including Australia (at Broken Hill), Germany, the United States (especially in Missouri, Kansas, and Oklahoma), and the United Kingdom . The mining and processing of galena remain critical industrial activities for lead production .
Synthesis Methods
In laboratory settings, Lead(II) sulfide can be synthesized through several methods. The most common approach involves the precipitation reaction between a soluble lead(II) salt and hydrogen sulfide or a sulfide source .
More sophisticated synthetic routes have been developed for specialized applications, particularly for producing high-quality nanoparticles. Research has shown that lead(II) complexes of thiosemicarbazone can serve as single-source precursors for the synthesis of Lead(II) sulfide nanoparticles with controlled morphologies . These methods typically involve thermal decomposition of the precursor compounds in the presence of capping agents such as hexadecylamine (HDA) or oleylamine (OLA) at temperatures ranging from 190°C to 270°C .
Industrial Production
Industrial processing of Lead(II) sulfide focuses primarily on extracting lead from galena ore rather than synthesizing the compound directly . The main industrial process involves a two-step method:
-
Smelting of PbS to produce lead(II) oxide and sulfur dioxide:
2 PbS + 3 O₂ → 2 PbO + 2 SO₂ -
Reduction of lead(II) oxide to metallic lead using carbon:
PbO + C → Pb + CO
The sulfur dioxide produced during this process is often captured and converted to sulfuric acid for industrial use, helping to reduce environmental impact . Modern production methods incorporate extensive pollution control measures to minimize the release of lead and sulfur compounds into the environment .
Applications and Uses
Semiconductor Applications
Lead(II) sulfide is a semiconductor material with a relatively narrow band gap, making it useful in various electronic applications . Its semiconductor properties have been known and utilized since the early days of semiconductor technology .
The semiconductor characteristics of Lead(II) sulfide make it suitable for use in transistors and other electronic components . Its narrow band gap, which can be tuned through nanoparticle size control, makes it particularly valuable for applications requiring specific electronic properties .
Recent research has focused on the development of Lead(II) sulfide quantum dots for use in solar cells, taking advantage of their tunable band gap and strong absorption properties . These quantum dots have shown promise for improving the efficiency of photovoltaic devices .
Photodetector and Infrared Sensing
One of the most significant applications of Lead(II) sulfide is in photodetectors, particularly for infrared radiation . PbS was one of the first materials used for electrical diodes capable of detecting electromagnetic radiation, including infrared light .
As an infrared sensor, Lead(II) sulfide functions as a direct detector of light, rather than as a thermal detector that responds to temperature changes caused by radiation . PbS detectors can operate in two modes: by measuring the photocurrent generated when photons strike the material, or by measuring the change in electrical resistance caused by the photons .
The increasing adoption of Lead(II) sulfide in infrared detectors across various industries, including defense, automotive, and security, is a key driver of market growth for this compound . These detectors are crucial components in night vision systems, motion sensors, and various scientific instruments .
Other Industrial Applications
Beyond its electronic and photodetector applications, Lead(II) sulfide serves various other industrial purposes:
-
High-temperature lubricants: The compound's physical properties make it suitable for use in specialized lubricants for high-temperature applications .
-
Ceramics and glazing: Lead(II) sulfide is used in glazing earthenware and in certain ceramic formulations .
-
Catalysis: It serves as a catalyst in petroleum refining, particularly for the removal of mercaptans from petroleum distillates .
-
Pigmentation: The black color of Lead(II) sulfide makes it useful as a pigment in specialized applications .
-
Slip property modifier: In certain materials, Lead(II) sulfide is utilized as a slip property modifier .
Recent Research and Developments
Nanoparticle Research
Research on Lead(II) sulfide has increasingly focused on nanoparticles and quantum dots, which exhibit unique size-dependent properties . Lead(II) sulfide nanoparticles have been well-studied and traditionally produced by combining lead salts with various sulfide sources .
Lead(II) sulfide nanoparticles have been synthesized with various morphologies, including cubic, rod-shaped, and nearly spherical forms . The synthesis methods typically involve the use of precursor compounds and capping agents to control growth and prevent agglomeration . Research has demonstrated that factors such as reaction temperature, capping group, and precursor type significantly affect the size, shape, and optical properties of the resulting nanoparticles .
Optical studies of Lead(II) sulfide nanoparticles reveal a blue shift in their absorption band edge compared to bulk material, with energy bandgaps ranging from 2.15 to 3.11 eV depending on particle size and synthesis conditions . This tunable bandgap makes these nanoparticles particularly interesting for optoelectronic applications .
Recent research examined Lead(II) sulfide nanoparticles for potential use in solar cells, leveraging their unique optical and electronic properties . The size of Lead(II) sulfide quantum dots can vary between 2.5 to 8 nm, resulting in emission wavelengths between 900-1600 nm, which covers a significant portion of the near-infrared spectrum .
Photocatalytic Applications
Emerging research has demonstrated the photocatalytic potential of Lead(II) sulfide nanoparticles, particularly for environmental remediation applications . Studies have shown that hexadecylamine (HDA)-capped Lead(II) sulfide nanoparticles can catalyze the degradation of methylene blue dye under UV irradiation, with degradation efficiencies ranging from 28.3% to 60.0% .
The highest photocatalytic efficiency (60.0%) was observed for Lead(II) sulfide nanoparticles obtained by thermolysis of thiosemicarbazone complex at 230°C . This finding suggests potential applications in wastewater treatment and environmental cleanup processes .
The photocatalytic activity of Lead(II) sulfide nanoparticles depends on several factors, including particle size, morphology, and surface properties . Optimizing these parameters through controlled synthesis methods represents an active area of research aimed at enhancing the photocatalytic performance of these materials .
Mass Molarity Calculator
- mass of a compound required to prepare a solution of known volume and concentration
- volume of solution required to dissolve a compound of known mass to a desired concentration
- concentration of a solution resulting from a known mass of compound in a specific volume