Iron selenide
CAS No.: 1310-32-3
Cat. No.: VC20948249
Molecular Formula: FeSe
Molecular Weight: 134.82 g/mol
* For research use only. Not for human or veterinary use.
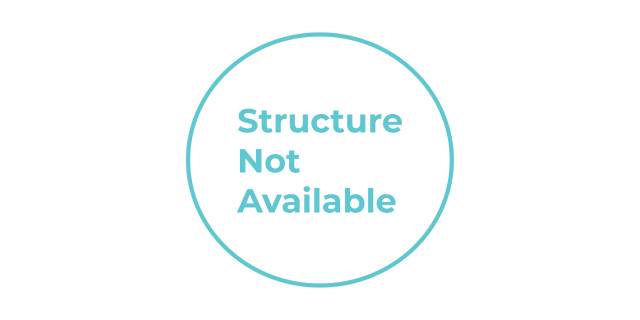
CAS No. | 1310-32-3 |
---|---|
Molecular Formula | FeSe |
Molecular Weight | 134.82 g/mol |
IUPAC Name | selanylideneiron |
Standard InChI | InChI=1S/Fe.Se |
Standard InChI Key | WALCGGIJOOWJIN-UHFFFAOYSA-N |
SMILES | [Fe]=[Se] |
Canonical SMILES | [Fe]=[Se] |
Chemical Properties and Structure
Iron selenide (FeSe) exhibits several notable physical and chemical properties. It appears as a black crystalline solid with a density of approximately 6700 kg/m³ . The compound decomposes upon heating rather than melting at a specific temperature, indicating its thermal instability under certain conditions .
The basic structural characteristics of iron selenide include:
Table 1: Basic Properties of Iron Selenide (FeSe)
Property | Value |
---|---|
Chemical Formula | FeSe |
Hill System Formula | Fe₁Se₁ |
CAS Registry Number | 1310-32-3 |
Formula Weight | 134.805 |
Physical Appearance | Black crystalline solid |
Density | 6700 kg/m³ |
Oxidation Number of Iron | +2 |
The elemental composition of iron selenide reflects its stoichiometric ratio: |
Table 2: Elemental Composition of Iron Selenide
Synthesis Methods
Nanocrystal Synthesis
Researchers have developed various approaches to synthesize iron selenide in nanocrystalline form. These methods offer control over morphology, crystallinity, and purity, which significantly influence the material's properties:
One established technique involves the one-pot thermal decomposition of ferrous chloride and selenium powder in oleylamine . This relatively straightforward method produces nanocrystals with controlled size and morphology.
More sophisticated approaches employ single molecular precursors. For example, researchers have synthesized iron selenide nanocrystals through the thermolysis of bis(tetraisopropyldiselenoimidodiphosphinato)iron(II) and bis(tetraphenyldiselenoimidodiphosphinato)iron(II) complexes in solvents like oleylamine and hexadecylamine . These methods allow for precise control of reaction conditions, resulting in rod and plate-like crystallites with defined morphologies .
Alternative synthesis routes include:
-
Elemental reaction in evacuated tubes at elevated temperatures
-
Reaction from aqueous metal salt solutions with H₂Se gas
-
Mechanical alloying techniques
-
Hydrothermal co-reduction methods using N₂H₄·H₂O as a reductant
-
Solvothermal methods producing various morphologies such as flower-like particles
Each synthesis approach results in iron selenide with specific characteristics, allowing researchers to tailor the material for particular applications.
Thin Film Deposition
The fabrication of iron selenide thin films represents an important area of research, particularly for electronic and superconducting applications. Aerosol Assisted Chemical Vapor Deposition (AACVD) has emerged as an effective technique for depositing iron selenide films on various substrates:
Researchers have successfully used bis(tetraisopropyldiselenoimidodiphosphinato)iron(II) and bis(tetraphenyldiselenoimidodiphosphinato)iron(II) complexes as precursors for AACVD onto silicon substrates . This approach allows for controlled deposition of thin films with tailored characteristics.
Interestingly, scanning electron microscopy (SEM) reveals that films deposited from different complexes display significantly different morphologies even under similar deposition conditions . This morphological diversity demonstrates the sensitivity of iron selenide's structure to its precursors and processing conditions, offering researchers multiple pathways to engineer films with specific properties.
Superconductivity in Iron Selenide
K-intercalated Iron Selenide
Researchers have discovered that chemical modification through potassium (K) intercalation can significantly enhance iron selenide's superconducting properties:
K-intercalated iron selenide (with nominal composition KxFe2-ySe2) demonstrates superconductivity above 30 Kelvin under ambient pressure conditions . This represents the highest superconducting transition temperature observed for FeSe-layer materials under ambient pressure at the time of discovery .
Hall effect measurements indicate that the charge carriers in K-intercalated iron selenide are predominantly electrons, providing crucial insights into the material's electronic transport mechanism . This intercalation approach effectively modifies both the structure and carrier concentration of iron selenide, demonstrating that FeSe-based materials can achieve remarkable superconducting properties through appropriate chemical and structural engineering .
Magnetic Correlations
Applications and Research Developments
Low-Coordinate Iron Selenide Complexes
Beyond the basic FeSe compound, researchers have synthesized and characterized various low-coordinate iron selenide complexes with unique properties:
Recent work has focused on the preparation of low-coordinate iron (poly)selenides resulting from the reaction of iron(I) silylamide with red selenium . These studies have yielded a variety of structures, including monoselenide bridged [2Fe-1Se]²⁺ complexes and mononuclear iron per- and triselenides .
Of particular interest is the observation of C-H bond activation by a putative terminal iron monoselenide, suggesting potential catalytic applications . These low-coordinate complexes serve as valuable structural models for surface sites in solid-state catalysts like FeSe₂, providing molecular-level insights into heterogeneous catalytic processes .
Catalytic Applications
Iron selenide materials have emerging applications in catalysis, particularly for energy-related chemical transformations:
Compounds such as FeSe₂ show promise as catalysts for hydrogen and oxygen evolution reactions, critical processes for renewable energy technologies . The surface heterogeneity of these materials under working conditions presents both challenges and opportunities for understanding the active sites responsible for catalytic activity.
Low-coordinate iron selenide complexes can function as structural models for different catalyst surface sites or even serve as precursors for synthesizing solid-state catalysts with tailored properties . This approach bridges fundamental coordination chemistry with practical applications in heterogeneous catalysis.
Mass Molarity Calculator
- mass of a compound required to prepare a solution of known volume and concentration
- volume of solution required to dissolve a compound of known mass to a desired concentration
- concentration of a solution resulting from a known mass of compound in a specific volume