S-Phosphocysteine
CAS No.: 115562-30-6
Cat. No.: VC20863905
Molecular Formula: C3H8NO5PS
Molecular Weight: 201.14 g/mol
* For research use only. Not for human or veterinary use.
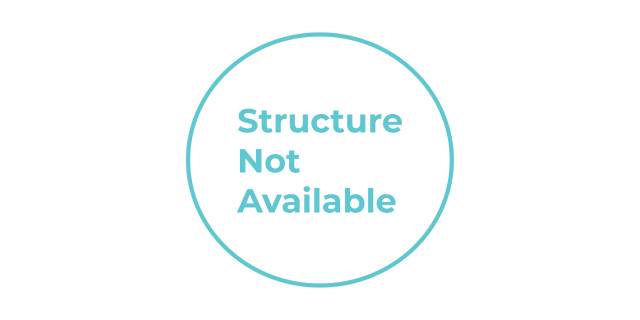
CAS No. | 115562-30-6 |
---|---|
Molecular Formula | C3H8NO5PS |
Molecular Weight | 201.14 g/mol |
IUPAC Name | (2R)-2-amino-3-phosphonosulfanylpropanoic acid |
Standard InChI | InChI=1S/C3H8NO5PS/c4-2(3(5)6)1-11-10(7,8)9/h2H,1,4H2,(H,5,6)(H2,7,8,9)/t2-/m0/s1 |
Standard InChI Key | MNEMQJJMDDZXRO-REOHCLBHSA-N |
Isomeric SMILES | C([C@@H](C(=O)O)N)SP(=O)(O)O |
SMILES | C(C(C(=O)O)N)SP(=O)(O)O |
Canonical SMILES | C(C(C(=O)O)N)SP(=O)(O)O |
Chemical Structure and Properties
S-Phosphocysteine (S-phospho-L-cysteine) is a phosphoamino acid consisting of L-cysteine carrying an S-phospho substituent. It belongs to the category of organic thiophosphates and S-substituted L-cysteine derivatives . The molecular structure features a phosphate group covalently bound to the sulfur atom of cysteine, forming a phosphorothiolate bond.
Molecular Identification and Basic Properties
S-Phosphocysteine possesses distinctive chemical characteristics that differentiate it from other phosphorylated amino acids. The compound's molecular formula is C3H8NO5PS with a molecular weight of 201.14 g/mol . Its systematic IUPAC name is (2R)-2-amino-3-phosphonosulfanylpropanoic acid, reflecting its stereochemistry and functional groups .
Table 1: Basic Chemical Identifiers of S-Phosphocysteine
Property | Value |
---|---|
Common Name | S-phosphocysteine |
IUPAC Name | (2R)-2-amino-3-phosphonosulfanylpropanoic acid |
CAS Number | 115562-30-6 |
Molecular Formula | C3H8NO5PS |
Molecular Weight | 201.14 g/mol |
ChEBI ID | CHEBI:22066 |
DrugBank ID | DB03544 |
Physical and Chemical Properties
S-Phosphocysteine exists as a solid at standard conditions with specific physical characteristics that influence its behavior in biological and chemical systems . The compound demonstrates particular thermal properties and chemical reactivity patterns, especially regarding the stability of its phosphorothiolate bond.
Table 2: Physical and Chemical Properties of S-Phosphocysteine
Property | Value |
---|---|
Physical State | Solid |
Density | 1.83 g/cm³ |
Boiling Point | 503.5°C at 760 mmHg |
Flash Point | 258.3°C |
31P NMR Chemical Shift | Approximately 12-14.8 ppm |
Bond Stability | Acid-labile phosphorothiolate bond |
Water Solubility | High (predicted) |
Hydrogen Bond Donors | 4 |
Hydrogen Bond Acceptors | 7 |
Polar Surface Area | 146 Ų |
A distinctive characteristic of S-phosphocysteine is the acid-lability of its phosphorothiolate bond, which presents challenges for its detection and isolation using standard phosphoproteomic approaches . This chemical instability differentiates it from other phosphorylated amino acids like phosphoserine or phosphothreonine, which form more stable phosphoester bonds. The phosphorothiolate bond's distinctive 31P NMR chemical shift, typically around 12-14.8 ppm, provides a useful spectroscopic signature for identifying this modification .
Biological Functions and Significance
S-Phosphocysteine has been identified in both prokaryotic and eukaryotic systems, suggesting evolutionary conservation of its functions across diverse life forms . Research has uncovered several critical biological roles for this modification, particularly in enzymatic reactions, cellular signaling pathways, and metabolite transport mechanisms.
Role in Phosphoenolpyruvate-Dependent Carbohydrate Transport
One of the most well-established functions of S-phosphocysteine is its involvement in the phosphoenolpyruvate (PEP)-dependent carbohydrate transport processes, particularly in bacterial systems. In these pathways, S-phosphocysteine residues in proteins function as key intermediates in phosphoryl transfer reactions .
The mechanism involves a sequential transfer of phosphoryl groups from phosphoenolpyruvate (PEP) through multiple proteins. Initially, the phosphoryl group is transferred to histidine residues of two cytoplasmic proteins, Enzyme I and HPr, which are common components across PEP-dependent carbohydrate transport systems. Subsequently, the phosphoryl group from HPr is transferred to essential histidine and cysteine residues located in the cytoplasmic domains of substrate-specific, membrane-bound transporter proteins .
In this cascade, both the N-phosphohistidine and S-phosphocysteine residues serve as transient catalytic intermediates facilitating the final phosphorylation of bound carbohydrate substrates . This phosphoryl transfer mechanism is fundamental to bacterial sugar uptake systems and represents a well-characterized example of S-phosphocysteine's functional significance.
Regulation of Protein-Protein Interactions and Magnesium Homeostasis
More recent research has uncovered a regulatory role for S-phosphocysteine in protein-protein interactions, specifically in the context of the PRL-CNNM pathway that mediates magnesium homeostasis. The formation of phosphocysteine within the protein tyrosine phosphatase PRL catalytic site modulates its interaction with CNNM magnesium transporters .
The phosphocysteine intermediate forms as part of the catalytic cycle and changes in response to magnesium levels in the cellular environment. Notably, phosphorylation of the cysteine residue blocks PRL binding to CNNM magnesium transporters, thereby affecting magnesium efflux . Mutations that disrupt the PRL-CNNM interaction have been shown to prevent regulation of magnesium efflux in cultured cells, highlighting the physiological significance of this phosphorylation-dependent mechanism .
This regulatory pathway has implications for energy metabolism and tumor progression, as magnesium is a critical cofactor in numerous enzymatic reactions and cellular processes . The discovery of phosphocysteine as a regulatory modification in this context opens new perspectives for understanding signaling mechanisms involving protein phosphatases.
Additional Biological Roles
Beyond its involvement in carbohydrate transport and magnesium homeostasis, S-phosphocysteine has been implicated in several other biological contexts:
-
It functions as an intermediate in the dephosphorylation of phosphotyrosine residues by protein tyrosine phosphatases .
-
S-phosphocysteine plays roles in bacterial signaling and regulatory pathways .
-
Recent research has explored the use of S-phosphocysteine as a mimetic for phosphoserine and phosphothreonine in certain experimental contexts .
These diverse functions underscore the biological versatility of S-phosphocysteine as a post-translational modification and suggest that its full range of roles in cellular processes may still be incompletely understood.
Detection and Analytical Methods
Mass Spectrometry-Based Approaches
Recent developments have addressed these challenges through specialized techniques. Electron-transfer higher-energy dissociation (EThcD) mass spectrometry has proven particularly effective for identifying and verifying phosphorylation on cysteine residues in peptides . This approach allows for the unambiguous assignment of phosphorylation sites, distinguishing phosphocysteine from other potential phosphoacceptors within the same peptide sequence.
The application of these advanced mass spectrometry techniques has facilitated the identification of endogenous cysteine phosphorylation sites in proteins such as IICB Glucose, a component of the bacterial phosphotransferase system (PTS) . These methodological advances promise to expand our knowledge of naturally occurring phosphocysteine modifications across different proteomes.
NMR Spectroscopy
Nuclear magnetic resonance (NMR) spectroscopy provides another valuable approach for analyzing S-phosphocysteine, particularly through 31P NMR observations. Phosphocysteine exhibits a characteristic chemical shift of approximately 14.8 ppm in 31P NMR spectra, similar to that reported for Enzyme II Mannitol (EIIMtl) .
Time-series NMR experiments have been used to monitor the formation and decay of phosphocysteine intermediates in protein phosphatases. For example, studies with PRL2 have shown that addition of suitable substrates leads to rapid formation of phosphocysteine, followed by its gradual decay and generation of free phosphate .
Additionally, two-dimensional NMR techniques such as 1H-31P HMBC (Heteronuclear Multiple Bond Correlation) experiments can confirm the attachment of phosphate to cysteine side chains by revealing coupling between the phosphorus signal and the α-methylene hydrogen atoms in the phosphocysteine side chain .
Enrichment Strategies and Other Analytical Approaches
The development of affinity enrichment methods for S-phosphopeptides has been an important focus in phosphoproteomic research. Current advances in phosphoproteomic methodologies provide increasingly adequate tools for investigating protein cysteine phosphorylation .
These methodological innovations appear immediately available for practical implementation in the emerging field of cysteine phosphoproteomics . Examples of methodological proposals for S-linked phosphorylation detection continue to stimulate the development of new approaches by the phosphoproteomic community, gradually overcoming the historical challenges associated with studying this modification.
Chemical Synthesis and Manipulation
The development of synthetic methods for producing S-phosphocysteine-containing peptides and proteins has been crucial for advancing research in this field. Recent innovations have focused on achieving chemoselective and stereochemically defined phosphorylation of cysteine residues.
Chemoselective Synthetic Methods
A significant breakthrough in this area has been the development of a chemoselective synthetic method to phosphorylate cysteine side chains in unprotected peptides . This approach leverages the reaction between nucleophilic phosphites and electrophilic disulfides, which can be prepared through standard methods .
The synthetic strategy typically involves:
-
Generation of peptides containing cysteine residues using standard solid-phase peptide synthesis (SPPS).
-
Conversion of cysteine to an activated disulfide, often using reagents like Ellman's reagent.
-
Reaction of the activated disulfide with suitable phosphite reagents to generate the phosphorothiolate ester.
-
Deprotection steps to yield the final S-phosphocysteine-containing peptide.
This methodology achieves the stereochemically defined phosphorylation of cysteine residues and has been validated through mass spectrometry and NMR analysis to confirm site-specific modification .
Alternative Approaches and Considerations
Other approaches for installing phosphocysteine include two-step methods using dehydroalanine (Dha) intermediates followed by reaction with sodium thiophosphate . While elegant, this strategy has limitations related to the harsh elimination conditions required to prepare Dha residues and the lack of stereoselectivity in the resulting phosphocysteine products.
The synthesis of phosphocysteine-containing peptides requires careful attention to reaction conditions and protective group strategies. For example, base-cleavable phosphite derivatives can lead to undesired side reactions, such as the formation of dehydroalanine during deprotection steps . These considerations have guided the development of refined protocols that minimize side reactions and maximize yields of the desired phosphorylated products.
The ability to synthesize defined phosphocysteine-containing peptides and proteins provides valuable tools for studying the biological functions of this modification, potentially enabling investigations into aspects of cysteine phosphorylation that have previously been inaccessible due to technical limitations.
Applications and Future Perspectives
The study of S-phosphocysteine has significant implications across multiple areas of biochemistry, molecular biology, and potential therapeutic applications. As analytical tools continue to improve, our understanding of this unique modification continues to expand.
Research Applications
S-phosphocysteine research contributes to fundamental understanding of protein post-translational modifications and their roles in cellular processes. The continued development of analytical methods for detecting and quantifying this modification will likely reveal its presence in previously unrecognized contexts.
Synthetic phosphocysteine-containing peptides provide valuable tools for studying enzyme mechanisms, particularly in phosphotransfer systems and protein phosphatases. These tools can help elucidate the structural and functional consequences of cysteine phosphorylation in various protein contexts.
Additionally, the emerging role of phosphocysteine in regulating protein-protein interactions, as demonstrated in the PRL-CNNM pathway, suggests that this modification may have broader significance in cellular signaling networks than previously appreciated .
Therapeutic Implications
The involvement of S-phosphocysteine in pathways related to magnesium homeostasis and tumor progression suggests potential therapeutic relevance. The PRL phosphatases, which form phosphocysteine intermediates as part of their catalytic cycle, have been identified as potential therapeutic targets .
Understanding the mechanisms by which phosphocysteine formation regulates protein-protein interactions could inform the development of targeted interventions for conditions involving dysregulated magnesium transport or PRL phosphatase activity.
Future Research Directions
Several promising areas for future investigation include:
-
Comprehensive proteome-wide profiling of S-phosphocysteine modifications across different organisms and physiological conditions.
-
Detailed mechanistic studies of phosphocysteine-mediated regulation in various biological pathways.
-
Development of specific inhibitors or modulators of phosphocysteine formation or recognition.
-
Investigation of potential crosstalk between cysteine phosphorylation and other post-translational modifications.
-
Further refinement of synthetic methodologies for site-specific incorporation of phosphocysteine into peptides and proteins with improved efficiency and scalability.
Mass Molarity Calculator
- mass of a compound required to prepare a solution of known volume and concentration
- volume of solution required to dissolve a compound of known mass to a desired concentration
- concentration of a solution resulting from a known mass of compound in a specific volume