Germanium selenide
CAS No.: 12065-11-1
Cat. No.: VC20740330
Molecular Formula: GeSe2
Molecular Weight: 230.6 g/mol
* For research use only. Not for human or veterinary use.
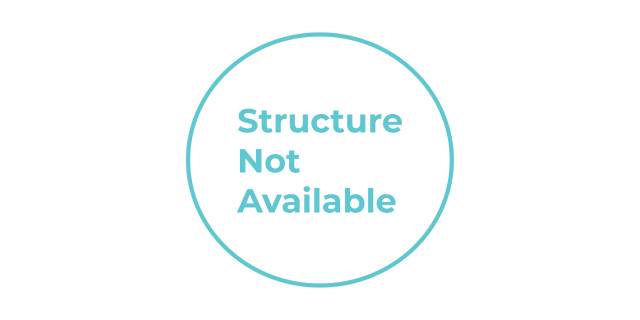
CAS No. | 12065-11-1 |
---|---|
Molecular Formula | GeSe2 |
Molecular Weight | 230.6 g/mol |
IUPAC Name | bis(selanylidene)germane |
Standard InChI | InChI=1S/GeSe2/c2-1-3 |
Standard InChI Key | WYDSCDJEAVCHQJ-UHFFFAOYSA-N |
SMILES | [Ge](=[Se])=[Se] |
Canonical SMILES | [Ge](=[Se])=[Se] |
Physical and Chemical Properties
Crystal Structure
Germanium selenide crystallizes in an orthorhombic structure with the space group D16-Pcmn (also denoted as Pnma in some literature) . Each unit cell contains four chemical units of GeSe. The crystal structure can be described as a distorted sodium chloride (NaCl) type structure, similar to tin sulfide (SnS) and tin selenide (SnSe) .
X-ray diffraction studies have revealed the precise unit cell parameters of GeSe crystals. The dimensions of the unit cell are:
Parameter | Value |
---|---|
a | 4.38 Å (0.438 nm) |
b | 3.82 Å (0.382 nm) |
c | 10.79 Å (1.079 nm) |
α, β, γ | 90° |
Table 1: Unit cell parameters of germanium selenide crystal
The atomic arrangement within the crystal results in Ge-Se bond distances of 2.54 Å, 2.58 Å, 3.30 Å, and 3.39 Å . This variation in bond lengths reflects the distorted nature of the crystal structure compared to the ideal NaCl structure. The GeSe layers are stacked together through van der Waals interactions, which are relatively weak compared to the covalent bonds within each layer . This structural characteristic enables the exfoliation of bulk GeSe into thin 2D layers, similar to other van der Waals materials.
GeSe crystals typically have a metallic appearance and can be grown with lateral dimensions of approximately 6-8 mm in rectangular shapes . High-purity GeSe crystals (>99.995%) can be synthesized and characterized using various techniques including X-ray diffraction (XRD), Raman spectroscopy, and energy-dispersive X-ray spectroscopy (EDX) .
Electronic Properties
Germanium selenide is a semiconductor with an indirect bandgap of approximately 1.1 eV, making it suitable for a wide range of electronic and optoelectronic applications . The electronic band structure of GeSe is characterized by multiple band extrema in close energy proximity within the first Brillouin zone . This feature contributes to its complex electronic behavior and anisotropic properties.
In its bulk form, GeSe demonstrates good electrical conductivity for a semiconductor material . The electronic properties are strongly influenced by the crystal orientation due to the material's inherent anisotropy. This directional dependence of electronic properties makes GeSe particularly interesting for applications requiring controlled conductivity paths.
When reduced to monolayer or few-layer thickness, the electronic properties of GeSe undergo significant modifications due to quantum confinement effects and reduced dielectric screening. The bandgap can be tuned by controlling the number of layers, offering a degree of flexibility in designing GeSe-based electronic devices .
One notable aspect of GeSe's electronic structure is its response to defects, particularly in two-dimensional forms. In monolayer GeSe, the presence of defects such as selenium vacancies creates localized "trap states" within the bandgap that strongly influence the electronic behavior of the material . These defects can significantly alter the electron and hole density distribution, leading to modified electronic and optical properties.
Optical Properties
The optical properties of germanium selenide make it particularly attractive for optoelectronic and photovoltaic applications. GeSe exhibits high optical absorption in the visible and near-infrared spectral regions, with a band gap ranging from 1 to 2 eV depending on the crystal structure and thickness .
A distinctive feature of GeSe's optical properties is their strong anisotropy. Polarization-resolved measurements have demonstrated a significant dependence of optical transitions on light polarization . This anisotropic behavior can be attributed to the material's orthorhombic crystal structure and the resulting directional electronic states.
Photogenerated current measurements of GeSe have shown reasonably high photoconversion efficiency and fast response times, indicating the material's potential for photodetection and solar energy conversion applications . The specific response characteristics depend on factors such as crystal quality, thickness, and the presence of defects or dopants.
In monolayer form, GeSe's optical properties are further modified by reduced dimensionality and quantum confinement effects. Studies have shown that selenium vacancies in monolayer GeSe can introduce sharp, low-energy optical absorption peaks below the optical gap of pristine material, resulting from strongly localized excitons .
Synthesis Methods
Powder Preparation
Several methods have been developed for the synthesis of germanium selenide powder, each with specific advantages for particular applications. One common approach involves the solid-state reaction of germanium and selenium powders under controlled conditions.
A detailed method for preparing GeSe powder involves the following steps:
-
Mixing germanium powder and selenium powder with a molar ratio of 1:1 using an agate mortar until homogeneous .
-
Transferring the mixture to a quartz or corundum crucible, which is then placed in a vacuum furnace .
-
Evacuating the furnace and replacing the gas with argon multiple times to ensure an inert atmosphere .
-
Heating the mixture to approximately 660°C at a rate of 3°C/minute under vacuum conditions and maintaining this temperature for a specified duration .
-
Cooling the reaction mixture and then pulverizing the product under argon gas protection to obtain the final GeSe powder .
For optimal results, the particle diameters of the starting germanium and selenium powders should be less than or equal to 500 nanometers . The briquetting of the powder mixture prior to heating can also improve the reaction efficiency and product homogeneity.
This method is advantageous for mass production due to its relatively simple process and readily available raw materials . The resulting GeSe powder can be further processed for various applications including thin films, electronic devices, and photovoltaic cells.
Applications
Photovoltaic Applications
The semiconductor properties of germanium selenide, particularly its bandgap of approximately 1.1 eV, position it as a promising material for photovoltaic applications . This bandgap value is close to the theoretical optimum for single-junction solar cells, allowing for efficient absorption of a substantial portion of the solar spectrum.
Recent advances in GeSe material development have focused on its application in thin-film solar cells (TFSCs) . The advantages of GeSe for solar cell applications include:
-
High optical absorption in the visible and near-infrared regions .
-
Bandgap in the optimal range for solar energy conversion (1-2 eV) .
-
Relatively abundant constituent elements compared to some other solar cell materials.
-
Demonstrated photoconversion efficiency and fast response time in experimental devices .
Photogenerated current measurements of GeSe-based devices have shown promising results, suggesting the material's potential for efficient solar energy conversion . The layered structure of GeSe also offers possibilities for creating heterostructures with other semiconductor materials to optimize band alignment and charge separation in solar cell devices.
Photoelectrochemical Applications
Beyond conventional photovoltaics, germanium selenide has shown potential for photoelectrochemical (PEC) water splitting applications . This technology aims to use solar energy to split water into hydrogen and oxygen, providing a clean and renewable method for hydrogen production.
The suitable band positions of GeSe relative to the water oxidation and reduction potentials, combined with its good light absorption and charge transport properties, make it a candidate material for photoelectrode applications in PEC cells . Research in this area is still developing, with various approaches being explored to optimize GeSe's performance for water splitting.
Theoretical studies, high-quality GeSe material synthesis, and device engineering efforts have contributed to advances in this field . The development of efficient PEC devices based on GeSe could contribute to sustainable hydrogen production technologies, addressing key challenges in renewable energy storage and utilization.
Recent Research Advances
Ultrafast Electron Dynamics
Recent research has provided insights into the ultrafast electron dynamics in germanium selenide, which is crucial for understanding and optimizing the material's performance in electronic and optoelectronic devices. Studies using core-hole clock spectroscopy have revealed that GeSe exhibits electronic states-dependent charge delocalization time .
Experiments involving resonant photoexcitation of core electrons to different final states have demonstrated that the delocalization time of electrons in high-energy electronic states probed from Se 1s is approximately 470 attoseconds (as), which is three times longer than the delocalization time of electrons in lower-energy electronic states probed from Ge 1s . This ultrafast timescale, in the sub-femtosecond range, highlights the extremely rapid electron processes occurring in GeSe.
These findings on energy-dependent dynamics in layered GeSe semiconductor offer opportunities for precisely distinguishing electron behavior at different energy levels. Such understanding could pave the way for designing ultrafast electronic devices with tailored response characteristics .
Defect Studies
The impact of defects on the properties of germanium selenide, particularly in its two-dimensional form, has been a focus of recent research. Studies combining density functional theory and many-body perturbation theory have investigated the effects of selenium vacancies on the electronic and optical properties of monolayer GeSe .
Research has revealed that selenium vacancies in monolayer GeSe result in midgap "trap states" that strongly localize electron and hole density . These defect states lead to sharp, low-energy optical absorption peaks below the predicted optical gap of pristine material. Analysis of the exciton wave function shows that the two-dimensional Wannier-Mott exciton characteristic of pristine monolayer GeSe is strongly modified by the presence of defects .
These findings have important implications for the design and optimization of GeSe-based devices. Understanding the role of defects allows for potential defect engineering approaches to tailor the material's properties for specific applications. Additionally, this research highlights the importance of defect control during material synthesis and device fabrication to achieve desired performance characteristics.
Mass Molarity Calculator
- mass of a compound required to prepare a solution of known volume and concentration
- volume of solution required to dissolve a compound of known mass to a desired concentration
- concentration of a solution resulting from a known mass of compound in a specific volume