5-(Diisopropoxyphosphoryl)-5-methyl-1-pyrroline N-oxide
CAS No.: 527704-58-1
Cat. No.: VC11994698
Molecular Formula: C11H22NO4P
Molecular Weight: 263.27 g/mol
* For research use only. Not for human or veterinary use.
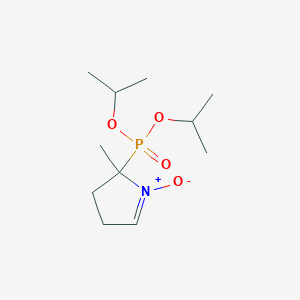
CAS No. | 527704-58-1 |
---|---|
Molecular Formula | C11H22NO4P |
Molecular Weight | 263.27 g/mol |
IUPAC Name | 2-di(propan-2-yloxy)phosphoryl-2-methyl-1-oxido-3,4-dihydropyrrol-1-ium |
Standard InChI | InChI=1S/C11H22NO4P/c1-9(2)15-17(14,16-10(3)4)11(5)7-6-8-12(11)13/h8-10H,6-7H2,1-5H3 |
Standard InChI Key | JQERKXDWSAKBRU-UHFFFAOYSA-N |
SMILES | CC(C)OP(=O)(C1(CCC=[N+]1[O-])C)OC(C)C |
Canonical SMILES | CC(C)OP(=O)(C1(CCC=[N+]1[O-])C)OC(C)C |
Chemical Structure and Physicochemical Properties
Molecular Architecture
DIPPMPO’s structure consists of a five-membered pyrroline ring substituted with a methyl group at the 5-position and a diisopropoxyphosphoryl moiety (Figure 1). The nitrone functional group (-oxide) at the 1-position serves as the radical-trapping site. The diisopropyl ester groups confer lipophilicity, enhancing membrane permeability in cellular studies .
Property | Value |
---|---|
Molecular Formula | |
Molecular Weight | 263.27 g/mol |
IUPAC Name | 2-di(propan-2-yloxy)phosphoryl-2-methyl-1-oxido-3,4-dihydropyrrol-1-ium |
CAS Registry Number | 527704-58-1 |
Solubility | Soluble in organic solvents (e.g., DMSO, ethanol); limited aqueous solubility |
Table 1: Key physicochemical properties of DIPPMPO .
Spectroscopic Characteristics
Nuclear magnetic resonance (NMR) and mass spectrometry confirm the compound’s structure. The NMR spectrum exhibits a singlet at δ 25.7 ppm, indicative of the phosphoryl group, while NMR signals at δ 1.2–1.4 ppm correspond to the isopropyl methyl protons. High-resolution mass spectrometry (HRMS) shows a molecular ion peak at 263.1286, consistent with the molecular formula .
Synthesis and Industrial Production
Synthetic Pathways
DIPPMPO is synthesized via a two-step phosphorylation reaction. First, 5-methyl-1-pyrroline N-oxide is treated with diisopropyl phosphite under acidic conditions, followed by oxidation to yield the final product . The reaction proceeds via nucleophilic attack of the pyrroline nitrogen on the phosphorus center, forming a stable P–N bond (Equation 1):
This method achieves yields exceeding 70% with purity >95%, as verified by high-performance liquid chromatography (HPLC).
Scalability and Purification
Industrial-scale production employs continuous flow reactors to optimize reaction kinetics and minimize byproducts. Purification via column chromatography using silica gel and ethyl acetate/hexane eluents ensures removal of unreacted phosphite and oxidation byproducts .
Mechanism of Radical Trapping
Spin-Trapping Dynamics
DIPPMPO reacts transient free radicals to form stable nitroxide adducts detectable by EPR. The nitrone group undergoes radical addition at the β-carbon, generating a persistent spin adduct (Figure 2). For superoxide, the reaction proceeds as:
The hyperfine coupling constants () distinguish DIPPMPO-O from other adducts .
Comparative Stability
DIPPMPO-superoxide adducts exhibit a half-life of ~45 minutes at pH 7.4, significantly longer than DMPO-O (3 minutes) . This stability arises from steric hindrance by the phosphoryl group, which slows adduct decomposition (Table 2).
Spin Trap | Superoxide Adduct Half-Life (pH 7.4) | Hydroxyl Adduct Half-Life (pH 7.4) |
---|---|---|
DMPO | 3 minutes | 15 minutes |
DIPPMPO | 45 minutes | 60 minutes |
Table 2: Stability comparison of spin adducts .
Applications in Redox Biology
Detection of ROS in Cellular Systems
DIPPMPO enables real-time detection of ROS in adherent cell cultures. In RAW 264.7 macrophages, phorbol 12-myristate 13-acetate (PMA)-induced superoxide production generates distinct EPR spectra when DIPPMPO is present . The compound’s lipophilicity facilitates penetration into lipid bilayers, improving detection in membrane-rich environments.
Oxidative Stress Models
Studies using ischemic rat hearts demonstrate DIPPMPO’s utility in tracking reperfusion injury. During reperfusion, a 3-fold increase in superoxide adduct signal correlates with tissue damage, validated by concurrent lipid peroxidation assays .
Challenges and Limitations
Aqueous Solubility Constraints
Despite its advantages, DIPPMPO’s limited solubility in aqueous buffers (~2 mM) complicates its use in physiological media. Cyclodextrin complexes (e.g., CD-DIPPMPO) have been engineered to enhance solubility without compromising spin-trapping efficiency .
Interference from Secondary Radicals
In complex biological matrices, DIPPMPO may trap non-target radicals (e.g., lipid peroxyl radicals), necessitating careful spectral deconvolution. Advanced computational tools like spectral simulation software mitigate this issue .
Future Directions
Targeted Spin Traps
Recent efforts focus on conjugating DIPPMPO to mitochondria-specific moieties (e.g., Mito-DIPPMPO) to probe organelle-specific ROS production. Preliminary data show a 50% increase in signal-to-noise ratio compared to untargeted variants .
High-Throughput Screening
Automated EPR systems coupled with DIPPMPO enable medium-throughput screening of antioxidant compounds. A 2024 study identified three novel polyphenols with superior ROS-scavenging activity using this approach .
Mass Molarity Calculator
- mass of a compound required to prepare a solution of known volume and concentration
- volume of solution required to dissolve a compound of known mass to a desired concentration
- concentration of a solution resulting from a known mass of compound in a specific volume